Marc R. Raffe1 and Elizabeth Goudie‐DeAngelis2 1 Veterinary Anesthesia and Critical Care Associates, LLC, St. Paul, Minnesota, USA 2 Tristate Veterinary Anesthesia, PLLC, White Plains, New York, USA The ideal anesthetic protocol for pregnant or periparturient (usually defined as labor and the time within a few weeks of labor) patients provides analgesia, muscle relaxation, and sedation/narcosis without endangering either mother or fetus. By their very nature, anesthetics, analgesics, tranquilizers, and sedatives cross the blood–brain barrier. The physicochemical properties that allow drugs to cross the blood–brain barrier also facilitate placental transfer. Factors in decision‐making regarding anesthesia protocols in these patients include the unique physiology of the mother during pregnancy and labor, the impact of selected drugs on the mother and fetus, carryover effects of administered drugs on the neonate following separation from the mother, and the effect of commonly observed anesthesia‐related complications on the fetus and mother. General anesthesia is needed for commonly performed periparturient procedures, but it is not possible to selectively anesthetize the mother because all agents that affect the maternal central nervous system will also affect the fetus. All drugs that change the maternal physiology will affect fetal homeostasis. This is especially pertinent during anesthesia for cesarean section when separation of the fetus from the uteroplacental attachment occurs. A complete understanding of the physiologic changes present in the gravid patient and the potential impact of anesthetic drugs is essential to navigate both mother and fetus safely through the perioperative period. Metabolic demands during gestation and parturition are met by physiologic adaptation, which is unique to this period (Table 45.1). Most of the data describing physiologic adaptation to pregnancy has been obtained from humans and ewes. Although little work has been done in other species, the changes should be comparable, if not greater, in magnitude because birth weight expressed as a percentage of maternal weight for people, sheep, dogs, and cats is 5.7%, 11.4%, 16.1%, and 13.2%, respectively [1]. This suggests that the physiologic burden and, therefore, physiologic adaptation may actually be greater in animals than humans. During pregnancy, maternal blood volume increases by approximately 40%; plasma volume increases by 40–50%, which is more than the 20% increase in red cell mass, resulting in decreased hemoglobin concentration and packed cell volume [2]. This results in a dilutional physiologic anemia. Increased heart rate and stroke volume cause cardiac output to increase 30–50% above normal [3,4]. Plasma estrogens decrease peripheral vascular resistance producing an increase in cardiac output, while systolic and diastolic blood pressures remain unchanged. Cardiac output is also influenced during labor by body position, pain, and apprehension [2]. During labor, systolic pressure increases by 10–30 mmHg. Cardiac output increases an additional 10–25% during labor and the immediate postpartum period, as a result of blood extrusion from the contracting uterus [5]. Although central venous pressure does not change during pregnancy, it increases slightly (4–6 cmH2O) during labor and has been reported to increase by up to 50 cmH2O during painful fetal extraction [6]. The posterior vena cava and aorta can be compressed by the enlarged uterus and its contents during dorsal recumbency. This can cause decreased venous return and cardiac output with concurrent reduction in uterine and renal blood flow [7,8]. Although this does not appear to be as serious a problem in dogs and cats, time spent restrained or positioned in dorsal recumbency should be kept to a minimum [7,8,9]. Table 45.1 Physiological alterations induced by pregnancy. Because cardiac work is increased during pregnancy and parturition, cardiac reserve is decreased. Patients with previously well‐compensated heart disease may suffer pulmonary congestion and heart failure caused by a gestation induced increase in cardiac workload and hemodynamic demand secondary to parturition‐associated pain [10]. In such patients, pain and anxiety control is a key component of successful patient management during the periparturient period. However, care must be taken to avoid additional cardiac depression and decompensation induced by excessive doses of sedatives or anesthetics. The use of ecbolic agents during or after parturition can adversely affect cardiovascular function. Oxytocin in large or repeated doses induces peripheral vasodilation and hypotension, which can adversely affect both mother and fetus through decreased tissue perfusion. Ergot derivatives used to control uterine bleeding can induce vasoconstriction and hypertension [11]. In humans, major changes to the coagulation system occur throughout pregnancy with the greatest changes noted at term [12]. There is often a mild, non‐clinically significant thrombocytopenia due to dilution, destruction, and consumption of platelets. The concentrations of clotting factors VII, VIII, IX, X, XI, Von Willebrand factor (VWF), and fibrinogen increase throughout pregnancy, while factors XI and XIII decrease, leading to a nearly doubling of coagulation activity at the end of pregnancy. Plasma fibrinolytic activity decreases until after placental delivery then returns to normal. There is a general increase in clotting ability at the time of parturition, which may increase the risk of perianesthetic clot formation in the dam. In humans, increased serum progesterone concentration during pregnancy increases respiratory center sensitivity to carbon dioxide. Increased minute volume during gestation produces a progressive reduction in PaCO2 during gestation; this value approaches 30 mmHg at parturition. Metabolic compensation for respiratory alkalosis maintains arterial pH within normal values. Hyperventilation may further be stimulated during labor by pain, apprehension, and anxiety. During pregnancy, oxygen consumption increases by 20% as a result of the demands imposed by the developing fetus, placenta, uterine muscle, and mammary tissue. Arterial oxygen tension remains unchanged [2]. Pregnancy also affects the mechanics of ventilation. Airway conductance is increased and total pulmonary resistance is decreased by progesterone‐induced relaxation of bronchial smooth muscle. Lung compliance is unaffected. Functional residual capacity (FRC) is decreased by displacement of the diaphragm and abdominal organs by the gravid uterus. In addition, FRC further decreases during labor because of increased pulmonary blood volume secondary to uterine contraction. Because FRC is reduced, small airway closure develops at end‐exhalation in approximately one‐third of human parturients [2]. Tidal volume increases by 45%, but total lung capacity and vital capacity are unaltered [13]. In addition, hypoventilation produces hypoxemia and hypercapnia more frequently in pregnant than non‐pregnant patients. Hypoxemia is exacerbated by increased oxygen consumption during labor. Oxygen administration prior to anesthetic induction increases oxygen reserve by facilitating pulmonary denitrogenation [14]. Preoxygenation is advisable if the patient is tolerant of this procedure. Induction of anesthesia with inhalation agents is more rapid in pregnant than non‐pregnant patients. The equilibration rate between inspired and alveolar anesthetic partial pressure is accelerated by increased alveolar ventilation via increased minute ventilation and decreased FRC. In the fetus, gas exchange occurs via the placenta. Carbon dioxide and oxygen move across the placental barrier by passive diffusion. Movement of carbon dioxide is facilitated by the Haldane effect; as the maternal blood releases oxygen to the fetus, its carbon dioxide carrying capacity is increased. As the fetal hemoglobin releases the carbon dioxide, its hemoglobin then becomes more able to bind to oxygen, referred to as the “double Haldane effect” [15]. Oxygen movement is facilitated by the Bohr effect. As maternal hemoglobin binds to carbon dioxide, the pH is decreased, which causes a right shift of the oxyhemoglobin dissociation curve. On the fetal side, the release of carbon dioxide from fetal hemoglobin results in a left shift of the oxyhemoglobin saturation curve, thus enhancing its binding affinity for oxygen; this is called the “double Bohr effect” [16]. In addition, fetal hemoglobin has a lower P50 than adult hemoglobin, enhancing its oxygen binding [17]. A number of functional changes in gastrointestinal physiology occur during gestation and parturition. Physical displacement of the stomach by the gravid uterus, decreased gastric motility, and changes in serum progesterone level contribute to delayed gastric emptying and are most pronounced during the last trimester. Acid, chloride, and enzyme concentrations in gastric secretions are increased due to changes in hormone physiology present during gestation. Lower esophageal sphincter tone is decreased, and intragastric pressure is increased. Pain and anxiety during labor have been shown to further decrease gastric motility [2]. These changes increase the risk of regurgitation (both active and passive), and aspiration is increased in parturients [18]. Frequently, patients presented for cesarean section have recently eaten or the time of the last feeding is unknown. Parturients should be regarded as having a full stomach, and anesthesia techniques should be selected that produce rapid airway management and control to prevent aspiration of foreign material. Because the risk of regurgitation and aspiration is considered high in periparturient patients, prophylactic measures are recommended. Metoclopramide and an H2‐receptor antagonist drug (e.g., cimetidine, ranitidine, or famotidine) may be administered as part of the preanesthetic protocol [19]. Prophylactic administration of antiemetics, such as maropitant and ondansetron, is a common practice in companion animals. Risk of vomiting increases with hypotension, hypoxia, and toxic reactions to local anesthetics. Smooth induction of general anesthesia and preventing hypotension during epidural anesthesia will decrease the incidence of vomiting. Because silent regurgitation can occur when the intragastric pressure is high, a cuffed endotracheal tube with adequate airway seal is preferred for airway management during general anesthesia. Passive regurgitation can be induced by positive‐pressure ventilation with a face mask or by manipulation of abdominal viscera. Although atropine reduces the lower esophageal sphincter (LES) pressure, it may decrease reflux by reducing the frequency of LES relaxation, but it may also inhibit the actions of metoclopramide that increase gastric motility and emptying by sensitizing gastric smooth muscle to acetylcholine [6,19]. The clinician should always be cognizant that gastroesophageal reflux can occur in the anesthetized patient (see Chapters 2 and 41 for further details). Pregnancy induces minor changes in hepatic function. Plasma protein concentration decreases slightly, but total plasma volume is increased because of the increase in blood volume. Bilirubin concentration is unaltered. Serum enzyme concentrations (serum alanine aminotransferase and alkaline phosphatase) can be slightly increased. Plasma cholinesterase (also known as pseudocholinesterase) concentration decreases, which may lead to prolonged action of succinylcholine in pregnant patients, particularly if they have been recently exposed to organophosphate parasiticides (e.g., anthelmintics, flea collars, or dips). Despite these alterations, overall liver function is generally well maintained [2]. Renal plasma flow and glomerular filtration rate are increased by approximately 60% in pregnant patients; blood urea nitrogen (BUN) and creatinine concentrations are lower than in non‐pregnant patients [6]. Sodium and water balance are unaffected. Elevated BUN or creatinine levels may indicate renal pathology or compromise in parturient patients. In these patients, drugs with known nephrotoxic potential, such as aminoglycoside antibiotics, and non‐steroidal anti‐inflammatory drugs (NSAIDs), should be avoided. Stable uteroplacental circulation is important to fetal and maternal homeostasis and neonatal survival. Uterine blood flow is directly proportional to systemic perfusion pressure and inversely proportional to vascular resistance in myometrial blood vessels. Placental perfusion is dependent on uteroplacental perfusion pressure, and there is no significant autoregulation in the uteroplacental circulation; however, placental vessels do have rudimentary mechanisms for changing vascular resistance and contain α‐adrenergic and β2‐adrenergic receptors [20]. Placental perfusion is directly linked to mean uterine perfusion pressure and uterine vascular resistance. Anesthesia may decrease uterine blood flow; in addition, uterine vascular resistance is increased by uterine contractions and hypertonia (oxytocic response). Placental hypotension is associated with hypovolemia, anesthetic‐induced cardiovascular depression, or sympathetic blockade resulting in reduced uterine perfusion pressure. Uterine vasoconstriction is induced by endogenous sympathetic discharge or by exogenous sympathomimetic drugs having α1‐adrenergic effects (e.g., epinephrine, norepinephrine, methoxamine, phenylephrine, and metaraminol) [2,20,21,22]. Hypotension induced by adjunctive drugs combined with increased uterine tone induced by ecbolics should be avoided. In humans, it has been reported that increased progesterone levels at term contribute to reduced anesthesia requirements. This observation may not be consistent in veterinary species; it is well‐documented in the dog and cat that progesterone levels decrease during gestation to basal, or slightly above basal, levels at term [23–27]. Horses have low blood progesterone levels at term; progesterone is undetectable in the blood during the second half of gestation as its source shifts from the corpus lutea in the ovary [28,29]. It is unlikely that progesterone has a significant impact on anesthesia requirements if cesarean section is warranted. The fetus’ capacity for gluconeogenesis is very limited. Therefore, as the fetal blood glucose source is from the mother, it is important to maintain normal maternal blood glucose concentrations. In the bitch, induction of labor results in a drop in core body temperature of at least 1.2 °C [23]. Pregnant (gravid) patients are at greater anesthetic risk because of pregnancy‐associated physiologic alterations. Cardiac reserve diminishes during pregnancy; high‐risk patients can suffer acute cardiac decompensation or failure. Gravid patients are prone to hypoventilation, hypoxia, and hypercapnia because of altered pulmonary function. The risk of embolism in the perianesthetic period is greater for these patients. Anesthetic requirements may be decreased, and the likelihood of a relative overdose and cardiorespiratory depression is greater than in non‐gravid patients. Emesis or regurgitation and aspiration can occur if induction of anesthesia is not immediately followed by rapid airway control. Pregnancy affects the uptake, distribution, and disposition of anesthetic agents and adjuncts. The concentration of free (non‐ionized, unbound) drug in maternal plasma is affected by changes in protein binding, placental transfer, distribution in fetal tissues, and biotransformation by the maternal and fetal liver. The effect of pregnancy on anesthetic agents has been studied [30]. The rate of barbiturate biotransformation appears to be decreased in pregnancy [30]. Succinylcholine and procaine metabolism are decreased because of decreased plasma cholinesterase concentration [30]. Increased renal blood flow and glomerular filtration associated with pregnancy favor renal drug excretion. In certain species, the minimum alveolar concentration (MAC) is reduced for all volatile agents [31]. MAC values are reduced in pregnant versus non‐pregnant ewes but not in canines [32]. Reduced MAC requirement coupled with a decreased FRC results in rapid anesthetic induction requiring as little as one‐fourth to one‐fifth of induction time compared to non‐pregnant patients [33]. Due to the increased rate of uptake, care must be taken to prevent volatile agent overdose in pregnant patients. The placenta is highly permeable to anesthetic drugs, and most anesthetics and anesthetic adjuncts diffuse quickly across the placental barrier. Placental transfer of drugs can occur by four mechanisms; the most important mechanism is simple diffusion (Fick’s law of diffusion). Other mechanisms include facilitated diffusion (glucocorticoids), active transport (norepinephrine and dopamine), and pinocytosis [33]. Diffusion across the placenta is determined by the drug’s molecular weight, the percentage of drug bound to maternal plasma protein, lipid solubility, and degree of ionization. Drugs with low molecular weight (MW < 500 Daltons [Da]), low degree of protein binding, high lipid solubility, and those that are non‐ionized at maternal blood pH diffuse rapidly across the placenta. Conversely, drugs that are larger (MW > 1000 Da), are highly protein bound, have low lipid solubility, and are mostly ionized generally cross the placenta slowly [30,33,34]. Neuromuscular blocking drugs, unlike other anesthetic and anesthetic adjunctive drugs, are highly ionized and have low lipid solubility. Although they can be recovered from fetal blood, they are generally regarded as having minimal placental transfer and negligible fetal effect [31,33]. The placenta does not appear to metabolize anesthetic drugs or adjuncts. Physiochemical properties within the fetus and mother also affect placental drug transfer [33]. The degree to which a drug is ionized is determined by its pKa and the pH of the maternal body fluids. Normal fetal pH is 0.1 unit less than that of the mother [30]. Drugs that are weak acids will be less ionized as pH decreases. For example, thiopental is a weak acid with a pKa of 7.6. In acidemic patients (pH < 7.4), a greater proportion of the administered dose is in the non‐ionized form. As the proportion of the non‐ionized form of the drug increases, the drug fraction that is protein bound is reduced, thus effectively increasing the clinical response on a milligram per kg basis. As a result, it is well recognized that acidemia decreases the required anesthetic dose of thiopental and other barbiturates. For weak basic drugs, lower fetal pH increases ionized drug concentration, thereby reducing the maternal–fetal concentration gradient and non‐ionized drug transfer across the placenta to the fetus. Weak basic drugs with pKa values near 7.4 such as opioids and local anesthetics are more highly ionized at pH values less than their pKa. Local anesthetics move across the placenta but do not easily diffuse back due to the lower pH of the fetus. Therefore, they are found in higher concentrations in fetal tissues and plasma compared to those of the mother because of “ion trapping.” Hence, their effect on the mother and fetus is less on a milligram per kg basis [30,33]. Fetal drug concentration is also affected by redistribution, metabolism, and protein binding. Drug concentration in the umbilical vein is greater than in fetal organs (brain, heart, and other vital organs). Approximately 85% of umbilical venous blood initially passes through the fetal liver where drugs may be sequestered or metabolized. In addition, umbilical venous blood containing drug enters the inferior vena cava via the ductus venosus and mixes with drug‐free blood returning from the lower extremities and pelvic viscera [30,33] (Fig. 45.1). Due to these mechanisms, it appears that fetal circulation buffers vital fetal tissues from sudden changes in drug concentration. Drug binding to fetal proteins may also reduce free‐drug availability [30,33]. Drugs dynamically redistribute out of the fetus into the mother as maternal plasma levels decrease, making clinical estimation of fetal plasma concentrations difficult. Additional variables in fetal drug concentration include slower drug metabolism contributing to a prolonged in vivo drug presence. Toxicity can be enhanced by fetal or maternal metabolism to more toxic metabolites and by drug–metabolite and drug–drug interactions [34]. Figure 45.1 The direction of blood flow in the fetal vascular system is indicated by arrows. The darkened vascular segments represent the umbilical blood and its path of flow into the liver and inferior vena cava via the ductus venosus. Blood flow through the foramen ovale and ductus arteriosus provides a direct path to the arterial system, bypassing the lungs. In neonates, the ductus arteriosus and foramen ovale close shortly after birth. This functional closure redirects blood flow through the neonate’s lungs where it is arterialized, as in the adult. The time required for complete anatomic closure of the foramen ovale varies by species and typically takes several months (but may require as many as 12 months in foals). Administration of a fixed dose of drugs with rapidly decreasing plasma concentration (e.g., thiopental, propofol, or succinylcholine) briefly exposes the fetus and placenta to a high maternal blood drug concentration. This is in contrast to the sustained maternal blood levels of drugs administered by continuous infusion or inhalation, which result in continuous placental transfer of drug to the fetus [30,34]. Maternal exposure to general anesthesia has potential consequences for the unborn fetus. Fetal risks change during development and are categorized by gestational trimester. General anesthesia during the first trimester of pregnancy is associated with fetal teratogenesis, spontaneous abortion, and fetal death. Anesthesia exposure during the middle trimester is generally considered the safest period of gestation; however, spontaneous abortion and fetal death have been reported during this period [35]. Anesthesia exposure during the last trimester carries a risk of premature labor and fetal death. There appears to be species variability with respect to anesthesia‐associated risk. This may be due to placental anatomy, uteroplacental perfusion, and maternal homeostasis. There does not appear to be a specific drug‐linked risk associated with increased fetal morbidity/mortality [35]. Care must be taken to ensure that maternal physiology is maintained during the anesthesia episode. Critical attention to support of maternal cardiorespiratory physiology, oxygen delivery, and acid–base balance ensures undisturbed uteroplacental perfusion and oxygen delivery; these are of paramount importance for postanesthetic fetal viability. In the human literature, it is recommended that NSAIDs be avoided after the first trimester to prevent premature closure of the ductus arteriosus [36,37]. Anesthetic drugs should be carefully chosen and properly administered to avoid excessive maternal depression and to maximize neonatal vigor and viability. As noted above, the specific characteristics that make a drug an excellent anesthetic agent are also those that facilitate rapid transplacental transfer and neonatal depression. Therefore, it is prudent to consider that no agent should be used unless distinctly indicated. A brief overview of anesthetic drug classes in periparturient anesthesia follows. Anticholinergic drugs, such as atropine and glycopyrrolate, have been historically used to decrease salivation and inhibit excessive vagal efferent activity that may occur when traction is applied to the uterus [38]. Their risk‐reducing value varies with species; they are more effective in dogs and cats, with less impact in other species. Glycopyrrolate may actually increase the viscosity of secretions via its drying properties, resulting in viscous saliva [39]. Many expectant mothers have eaten immediately before delivery. The presence of gastric contents increases the likelihood of regurgitation, which is enhanced by hypoxia or hypotension. The influence of anticholinergics upon emesis is variable [6,38]. In women, atropine has not been shown to decrease the incidence of emesis at parturition [34]. Glycopyrrolate has been shown to be equivalent to ondansetron in prevention of postoperative nausea and vomiting [39]. Additionally, because glycopyrrolate does not readily cross the placenta, it does not affect the fetus to the same extent as atropine. Because of their long duration of action, there is no indication for the routine use of these agents in parturients [6,33]. They should be restricted to markedly apprehensive or excited patients and only in doses sufficient to induce a calming effect. Acepromazine can induce significant maternal and fetal depression even at relatively low doses. Diazepam and midazolam can induce neonatal depression characterized by the absence of vocalization, lethargy, hypotonus, apnea, and hypothermia immediately following birth [40–42]. It has been suggested that these effects are dose related and can be minimized by administering low doses of diazepam (< 0.14 mg/kg IV), although no safe dose has been established in domestic animals [42]. Residual benzodiazepine‐induced lethargy and muscle relaxation in either the mother or neonate can be antagonized with flumazenil, a specific benzodiazepine antagonist administered to effect [43]. α2‐Adrenergic receptor agonists have significant effects on both maternal and fetal homeostasis. Xylazine rapidly crosses the placenta and induces both maternal and fetal respiratory and circulatory depression. When used with ketamine, xylazine produces significant cardiopulmonary changes, resulting in decreased tissue perfusion in healthy dogs [44]. The use of xylazine or xylazine–ketamine combinations should be avoided in small animal patients presented for cesarean section [45]. However, combinations of xylazine and ketamine have often been used in mares presenting with dystocia. Detomidine appears to be well tolerated when administered to pregnant mares at 20–60 μg/kg IV. Two studies demonstrated no increase in drug‐related change in uterine activity or increased incidence of spontaneous abortion following repeated dosing [46,47]. Similar results have been reported in cattle [48]. Limited information is available regarding the effect of medetomidine on pregnancy. In dogs, there appears to be a dose‐related effect on uterine muscle activity that was documented during the parturient and immediate post‐parturient period [49]. A study in which medetomidine was used as a premedicant in canine cesarean section reported a high percentage of viable puppies following surgery [50]. All puppies and bitches were prophylactically administered atipamezole following delivery. Similar results were reported when dexmedetomidine was used as a premedicant in canine cesarean section [51]. There are case reports of dexmedetomidine use in human cesarean section patients with good success [52]. Romifidine induces a transient increase in intrauterine pressure following administration [53]. There is very little information on trazodone use in pregnancy; one small multicenter study evaluating trazodone use in humans during both the first trimester and throughout gestation did not find a higher incidence of major malformations, but there may have been an increase in spontaneous abortion [54]. There were no data on neonatal vigor within this study. More data are available on gabapentin; exposure both early and late in gestation resulted in cardiac defects, increased risk of preterm birth, and increased need for neonatal intensive care [55]. Gabapentin is minimally secreted into milk with no side effects noted in suckling infants. Similarly, studies of infants whose mothers were taking trazodone during breastfeeding found very low levels within milk and few side effects in the infants [56]. Both trazodone and gabapentin should be used with caution during gestation and only as needed in the periparturient period. Opioids rapidly cross the placenta and can cause neonatal respiratory and neurobehavioral depression [34,57,58]. In addition, fetal elimination may require 2–6 days. It appears that equianalgesic doses of opioids induce an equal degree of central nervous system depression. Therefore, choice is based on the desired duration of opioid action. Commonly used μ‐opioid receptor agonists include morphine, fentanyl, meperidine, oxymorphone, hydromorphone, and methadone [34]. Agents having opioid agonist–antagonist or partial agonist activity include butorphanol and buprenorphine, respectively. Butorphanol provides mild to moderate sedation but less intense analgesia than a full agonist. Buprenorphine produces mild sedation plus good to excellent analgesia.
45
Anesthetic Considerations During Pregnancy and for the Newborn
Introduction
Pregnancy‐associated changes in maternal physiology
Cardiovascular
Variable
Change
Heart rate
↑
Cardiac output
↑
Blood volume
↑
Plasma volume
↑
Packed cell volume, hemoglobin, and plasma protein
↓
Arterial blood pressure
unchanged
Central venous pressure
unchanged; ↑ during labor
Minute volume of ventilation
↑
Oxygen consumption
↑
pHa and PaO2
unchanged
PaCO2
↓
Total lung and vital capacity
unchanged
Functional residual capacity
↓
Gastric emptying time and intragastric pressure
↑
Gastric motility and pH of gastric secretions
↓
Gastric chloride ion and enzyme concentration
↑
AST, LDH, and BSP retention time
↑
Plasma cholinesterase
↓
Renal plasma flow and glomerular filtration rate
↑
Blood urea nitrogen and creatinine
↓
Sodium ion and water balance
unchanged
Coagulation
Pulmonary
Gastrointestinal
Liver and kidney
Uterine blood flow
Neuroendocrine
Summary
Pharmacologic alterations induced by pregnancy
Anesthesia during pregnancy
Anesthetic drugs and cesarean section
Anticholinergics
Tranquilizers and sedatives
Opioids
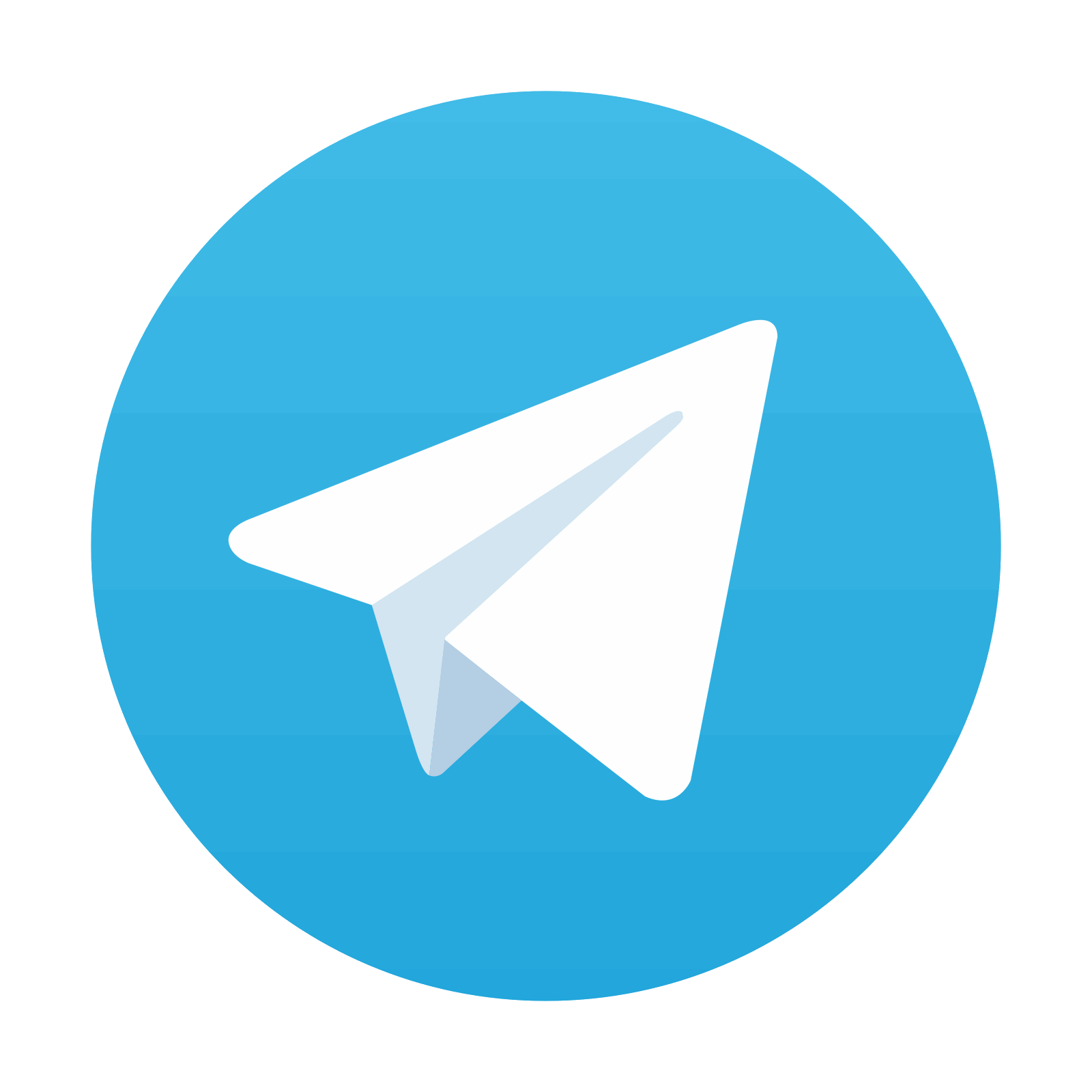
Stay updated, free articles. Join our Telegram channel

Full access? Get Clinical Tree
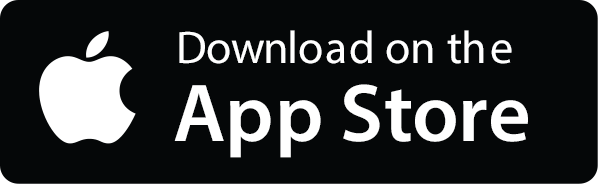
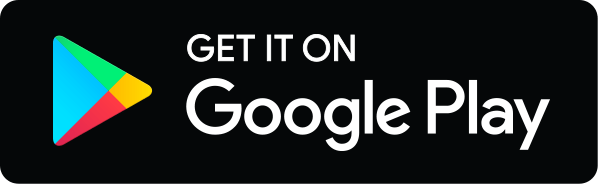