Khursheed R. Mama Department of Clinical Sciences, College of Veterinary Medicine and Biomedical Sciences, Colorado State University, Fort Collins, Colorado, USA The term “cardiopulmonary bypass” is broadly applied to the procedure by which blood is diverted away from the heart and lungs while also providing oxygenated blood to other organs to sustain viability. There is a long history of the use of animals, predominantly pigs and sheep, for cardiovascular surgical training and research. Additionally, over the past three decades, animals, most notably dogs (albeit there are occasional reports of cats [1]), have been anesthetized with varying frequency in the clinical arena to facilitate surgical procedures involving the heart (and lungs) [2–15]. Although perioperative morbidity is common (e.g., dysrhythmias, bleeding, phrenic nerve damage, hypoventilation, and hypoxemia), acute survival (off pump to recovery) percentages from the aforementioned reports are generally high, even 100%. Longer‐term survival, however, is variable, with wide‐ranging complications including hemorrhage, thromboembolism, pleural effusion, heart failure, and sudden death being reported. The focus of this chapter is to introduce the reader to cardiopulmonary bypass as used to facilitate cardiac surgery in canine patients. Challenges associated with this as a clinical tool have been reviewed [16]. Although anesthesia management is reviewed here, much of the chapter is devoted to introducing the basics of circuitry and instrumentation used to facilitate cardiopulmonary bypass and the considerations (e.g., cardiac protection and management of coagulation) that arise as a result. As the reader is no doubt aware, many cardiac procedures may be performed on the beating heart, sometimes with the added utilization of inflow occlusion and hypothermia. This chapter is limited to a discussion of the management of clinical canine patients where cardiopulmonary bypass is necessary to facilitate cardiac surgery. The circuit external to the patient consists of a reservoir for blood and fluids used to prime the pump, a membrane (versus bubble) [17] oxygenator of appropriate size (pediatric size is commonly used for small‐ to medium‐sized dogs), ventilating system, pumps (roller or centrifugal) [18,19] of varying number (three to five) to facilitate circulation of blood, a heat exchanger, and circulating heating/cooling water bath to permit initial cooling and subsequent warming of the patient (Fig. 34.1). Tubing appropriate to the patient’s size (so that excessive hemodilution resulting from excessive prime volume, exposure to circuit components, and subsequent red blood cell trauma can be minimized) connects these parts and is attached to lines from/to the patient. As the lung is no longer functional during this process, an oxygen flowmeter (or oxygen and air flowmeters along with an oxygen blender to vary the oxygen fraction) and vaporizer are connected to the circuit to facilitate the delivery of inhaled anesthetic as needed. Maintenance of anesthesia with parenterally administered injectable drugs has also been described [14]. Newer bypass circuits have an independent pump and tubing mechanism for the delivery of cardioplegic solutions, arterial and/or venous in‐line monitors (to record temperature, hematocrit, saturation, etc.), and safety devices (e.g., bubble detector and flow sensors). A more complete description and diagram of bypass circuitry available for human cardiopulmonary bypass are available in other medical texts [20]. Figure 34.1 Standard and pediatric cardiopulmonary bypass pumps. Although the personnel responsible for the anesthetic management of the patient should have a general understanding of the external circuitry used, the author’s experience has been that an individual with specialized training in perfusion should be responsible for ensuring functionality of the external circuitry and bypass equipment. The anesthesia personnel will, however, need to be in constant communication and provide the perfusionist with patient‐specific information, such as the hematocrit and calculated blood volume. This information will be used to determine the pump prime volume with a target hematocrit of 25–30% to optimize capillary flow and oxygen‐carrying capacity during bypass when hypothermia causes an increase in blood viscosity [21]. The perfusionist may request crystalloid fluids, synthetic colloids, blood, plasma or packed red cells (usually in smaller patients), and additives such as mannitol, sodium bicarbonate, and heparin to add to the pump prime. Steroids are added to the pump prime in some circumstances but with decreasing frequency. The perfusion team may also request a vaporizer and inhalant anesthetic consistent with what the anesthesia team is using for the patient. Additional details regarding types and sizes of oxygenators, calculation of pump prime volume, components of prime solution, types and sizes of tubing, etc. may be found elsewhere [21–23]. Patient cannulation is performed by the surgical team. A lateral thoracotomy is the preferred approach in the dog if the cardiac procedure to be performed allows for this; a median sternotomy approach is a feasible alternative. Commonly, arterial (oxygenated) blood is actively returned (using a pump) to the patient via a cannula placed in the femoral or carotid artery. It is important that the anesthesia team is aware of which site will be used and, specifically for the femoral vessels, which limb will be utilized, so they do not place the arterial catheter to monitor systemic arterial blood pressure in the same limb. The arterial cannula is either placed first or at the same time as the thoracotomy is performed if there are two surgeons. Early placement facilitates a quicker connection to the bypass circuitry if the patient’s status deteriorates during placement of the venous cannula(e). Figure 34.2 Bicaval cannulation for removal of a right atrial mass (in the center of the picture). The caudal vena cava is in the 11 o’clock position and cranial vena cava in the 1 o’clock position. Tape ties are pulled up around each cava, to prevent blood leakage into the right atrium. The cardiotomy sucker in the 7 o’clock position is in place to drain any coronary sinus flow. Bicaval or atrial venous cannulation is performed by the surgical team depending on the procedure to be performed. For procedures involving the right heart, bicaval cannulation is preferred to minimize blood in the surgical field and minimize compromise to venous drainage if the heart is retracted during the procedure (Fig. 34.2). The venous line connected to the cannula passively drains the majority of blood returned to the heart into the reservoir of the bypass circuit. Blood returning from the thebesian veins, coronary sinus, and systemic collaterals can usually be drained by appropriate (depending on the nature of the surgery) placement of these cannulas to reduce the risk of right ventricular distention. As this is a gravity‐dependent process, the height of the patient above the reservoir is an important consideration. Suction may be cautiously applied to venous drainage if needed. An additional left ventricular vent line may be placed to minimize left ventricular distention and warming resulting from drainage of systemic, pericardial, and bronchopulmonary collaterals and thebesian veins [20]. A surgical suction cannula is also available and may be connected with a line to the reservoir. Placement of the aforementioned cannulas facilitates extracorporeal circulation and a bloodless surgical field but does not offer a mechanism for myocardial protection. An additional cannula is, therefore, placed for administration of cardioplegia. This cannula may be placed such that flow of cardioplegic solution is anterograde via an aortic root cannula located on the cardiac side of the aortic cross clamp, or retrograde via a balloon catheter in the coronary sinus. Cardioplegic solutions are administered to stop cardiac activity once the aortic cross clamp is placed to reduce energy requirements, protect myocardial cells, and scavenge free radicals. Cardioplegic solutions may be blood‐based (sanguineous) or crystalloid‐based, with additives for cardiac protection (e.g., mannitol or lidocaine) and high levels of potassium to stop electrical activity. Sanguineous solutions are thought to provide better oxygen delivery and scavenging of free radicals, especially for longer procedures [20,23]. Additional myocardial protection is achieved using systemic and/or local hypothermia (using iced saline slush around the heart if appropriate and with adequate protection of the phrenic nerve). Although cardiopulmonary bypass offers ideal conditions for surgical intervention, the processes involved also generate real or potential negative effects on a multitude of organ systems. These have been reviewed extensively and many continue to form the basis of ongoing study [24–27]. Briefly, contact of blood components with the cardiopulmonary bypass circuitry (tubing, pump, and oxygenator) rapidly results in significant activation of the inflammatory cascade not dissimilar to that occurring in sepsis. Surgical processes also contribute and together these result in platelet, white blood cell, complement, and cytokine activation. The consequences of this inflammatory response can be far reaching, influencing coagulation, which, in turn, can increase microemboli and bleeding and result in organ dysfunction. This is, therefore, an area of significant investigation both into how anesthetic drugs or/and modifications in circuit components (e.g., heparin‐bonded circuits) might favorably modulate these responses to benefit the patient. A few of the common medications and strategies that aim to maximize patient outcome in light of these changes are presented in the next section. Owing to the invasive nature of the surgical procedure and inflammatory changes resulting from bypass, a broad‐spectrum antibiotic is administered prior to and at fixed intervals during surgery. Historically, because of the many cellular and humoral changes that occur during cardiopulmonary bypass, steroid use has been advocated. Although steroid administration is associated with an increase in blood glucose, which, in turn, is associated with a poor neurologic outcome, considerations such as improved hemodynamic stability, lower rates of postoperative atrial fibrillation, and reduced myocardial injury and inflammation have generally outweighed concerns [28–30]. More recently, while use may still be advocated in children even though evidence of benefit is limited [31], steroid use is considered equivocal in adults with some studies suggesting increased risk of myocardial infarction and lack of benefit to outcome. A meta‐analysis suggests that dose may have a significant impact on results following steroid use with low doses being considered potentially beneficial and high doses potentially detrimental [32]. Steroids are no longer routinely administered to dogs prior to cardiopulmonary bypass at the author’s institution. Both lysine analogs (e.g., ε‐aminocaproic acid and tranexamic acid) that inhibit proteases through antagonism of free plasmin, and nonspecific protease (or plasmin) and kalikrein inhibitors (e.g., aprotinin) have been used preoperatively with to the aim of reducing the need for post‐bypass transfusion in human patients [33–35]. The lysine analogs act as antifibrinolytics and help prevent dissolution of the clot, while aprotinin has both antifibrinolytic and anti‐inflammatory actions. While aprotinin was used in both human and canine patients and was effective at reducing transfusion requirements perioperatively, there was suggestion of increased risk of renal, neurologic, and cardiovascular complications and anaphylaxis, which led to its (temporary) withdrawal. Both tranexamic and aminocaproic acid continue to be used in human patients although dosing guidelines (dosage and timing of administration) vary. Seizures are more commonly reported with tranexamic acid as compared to aminocaproic acid but both are effective at decreasing the need for transfusions in people [36]. Our current practice is to use ε‐aminocaproic acid prior to and during cardiopulmonary bypass and until chest wall closure. Following assessment of coagulation parameters and clinical signs, additional drug may be administered during the postoperative period. Heparin is approved and routinely utilized in human patients for anticoagulation during cardiopulmonary bypass despite the large individual variability in its actions. An initial dose of 300–400 IU/kg of unfractionated heparin has been recommended for human patients and has also been used in clinical canine patients. In the author’s experience, the activated clotting time (ACT) following administration of this dose to dogs is typically greater than 1000 s when blood is sampled approximately 10 min after administration; an ACT greater than 400–480 s (in hypothermic patients) is considered by most to be adequate to initiate bypass. Repeated (often partial) dosing is considered necessary if ACT values approach this, while the patient is still connected to the bypass circuit. With the availability of heparin‐coated circuits, both initial and repeat dosing may need to be adjusted. Low‐molecular‐weight heparin (e.g., dalteparin and enoxaparin) and other anticoagulants that result in direct Factor IIa (thrombin) inhibition (e.g., argatroban and dabigratran), in addition to antiplatelet medications (e.g., clopidogrel) and factor Xa inhibitors (e.g., apixaban and rivaroxaban), are increasingly available for use in the management of (anti)coagulation in humans. Some of these have been studied and used in dogs and cats, but specific studies of their use during cardiopulmonary bypass are lacking [37–40]; however, they are often used for weeks to months postoperatively to prevent clot formation. The effects of heparin are reversed with protamine sulfate at the conclusion of cardiopulmonary bypass. As discussed later, slow administration is advised as protamine is reported to cause significant hypotension in dogs when administered rapidly [41]. This is a strategy commonly used during cardiopulmonary bypass to reduce metabolic rate and oxygen consumption. Hypothermia preserves high‐energy phosphate stores and reduces excitatory neurotransmitter release, calcium‐mediated enzyme induction, and cell destruction [42,43
34
Anesthesia for Cardiopulmonary Bypass
Introduction
The circuitry and patient cannulation
The external circuit
Patient cannulation
Systemic responses – pathobiology
Drugs and interventions used during cardiopulmonary bypass
Antibiotics
Steroids
Antifibrinolytics
Anticoagulation and reversal
Hypothermia
Stay updated, free articles. Join our Telegram channel

Full access? Get Clinical Tree
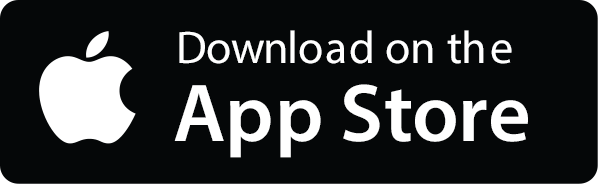
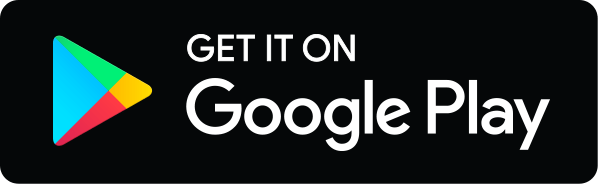