Fig. 15.1
Severe aortic valve stenosis of CAWS arteritis in DBA/2 mice
CAWS treatment DBA/2 mice were administered with CAWS via peritoneal injections for five consecutive days
(a) Untereated aortic valve of DBA/2 mouse, (b) The deformed aortic valve at fourth week after CAWS administration in DBA/2 mouse
The characteristics of inflammatory cells in CAWS vasculitis and how they affect the microstructure of the arteries were investigated in detail. Elastica van Gieson (EVG) staining revealed destroyed tunica media elastic lamina and inflammatory cells directly infiltrating into the extracellular matrix structure. Furthermore, 2 weeks after CAWS administration, infiltration of granulocyte cells through the elastic fibers was observed in vasculitis lesions. Inflammatory cells of various origins were observed, partly forming an abscess, and the infiltration of granulocytes abundantly containing granules within the cells, histiocytes filled with nuclei, and lymphoid cells was observed. These findings clearly showed that, in DBA/2 mice, CAWS vasculitis is characterized by marked infiltration and accumulation of various inflammatory cells, leading to the destruction of the basic three-layer structure of the arteries [22].
In addition to the name and definition of vasculitis according to the classification of Fauci et al., the Chapel Hill Consensus Conference (CHCC) proposed a vasculitis classification in 1994 [23]. According to the CHCC classification, in which vasculitis is classified on the basis of the size of vessels containing lesions, some forms of vasculitis show lesions in large vessels such as the aorta (giant cell arteritis or temporal arteritis, Takayasu’s arteritis), whereas other forms show lesions in small- to medium-sized arteries that branch into each organ or tissue (polyarteritis nodosa, KD, Wegener’s granulomatosis, and Churg-Strauss syndrome). Other forms accompanying capillary inflammation include Henoch-Schonlein purpura and hypersensitivity vasculitis. Various clinical symptoms are presented depending on the site of the blood vessel affected. Almost 20 years has passed since the establishment of the classification, and with discussions regarding the handling of ANCA-related vasculitis among others, a new classification based on international clinical studies (DCVAS) [24] and a revised version of CHCC (CHCC 2012) have recently been proposed [25]. Regarding CAWS vasculitis observed in this study, inflammatory lesions found in all layers and over the entire circumference are observed in the aortic root as well as in the proximal portion of the coronary arteries. Thus, in terms of the site of lesion formation, CAWS vasculitis resembles KD. From the viewpoint of how inflammatory lesions develop over time, CAWS vasculitis shares more similarities with giant cell arteritis or Takayasu’s arteritis, which develops from the adventitial side, as the accumulation of inflammatory cells toward the adventitial side is observed in the earliest stage, with almost no formation of thrombus (which strongly suggests desquamated endothelial cells and intimal damage). Histologically, however, CAWS vasculitis is classified as necrotizing vasculitis, since the basic vessel structure is destroyed 3 weeks after CAWS administration, and fibrinoid necrosis of the medial elastic fibers and intimal thickening are observed [26]. Inflammatory tissue is composed primarily of polynuclear leukocytes, and as inflammatory cells accumulate in the adventitia, migrate, and invade into the vasa vasorum, the infiltration progresses along the elastic fibers of the tunica media, possibly expanding inflammatory lesions [27, 28].
Although the localized nature of vascular lesions has not been fully elucidated, the involvement of anti-aortic antibodies (autoantibodies responsive to endothelial cells) in Takayasu’s arteritis and immune complexes and antineutrophil cytoplasmic antibodies (ANCAs) in vasculitis of the small- and medium-sized vascular system has been suggested [26]. Thus, it is possible that there is a dependence on the type of autoantigen. Moreover, given that the formation of vascular lesions, including atherosclerosis, often occurs in branched or curved areas of the arteries, physical stimuli in the vascular tissue, such as shear stress at branch points and sudden changes in wall pressure and blood flow, might be related to endothelial cell damage, with possible involvement in the localized nature of the lesions [29].
CAWS vasculitis represents an inflammatory response accompanied by significant enlargement of the spleen, and in vitro CAWS stimulation has been reported to increase inflammatory cytokine production markedly in splenocytes of DBA/2 mice [12]. The incidence and lethal toxicity of CAWS vasculitis, as well as its cytokine-inducing ability, greatly vary between different mouse strains [10–14, 19]. Associations between some forms of vasculitis and HLA alleles in humans have been suggested [30], but how the H-2 allele is associated with vasculitis in mice has not been examined fully. Moreover, DBA/2 mice are known to develop epicardial calcification frequently, which has been reported to be attributable to their abnormal nutritional status [31]. Since evidence of calcification is interpreted as the final outcome of inflammatory responses, the possibility cannot be ruled out that DBA/2 mice are susceptible to chronic inflammation of the vascular system.
In an animal model of KD-like vasculitis, vasculitis that resembles KD [32] can be caused by not only fungus-derived substances such as CAWS and CADS but also bacterium-derived substances such as Lactobacillus casei cell wall extracts (LCWE/LCCWE). In recent years, many reports have suggested the involvement of microbial infection and microbial antigens as a cause of vasculitis. These include the involvement of viruses such as human immunodeficiency virus, parvovirus B19, cytomegalovirus, and varicella-zoster virus and the involvement of infections by bacteria such as Staphylococcus aureus [33]. With the development of research on innate immunity, immunoactivating substances derived from microorganisms are now collectively referred to as PAMPs [34]. PAMPs are recognized by the innate immune system and control the differentiation of T cells through the activation of dendritic cells [35]. Many immunostimulants generally possess the characteristics of PAMPs, and although immunostimulants cause fewer side effects, various effects of PAMPs that stimulate immune responses could result in adverse events. It is worth noting that the murine model of CAWS vasculitis provides data suggesting this possibility.
Apo-E KO mice have been used widely as an animal model of atherosclerotic lesions. In Apo-E KO mice, arterial lesions are reported mainly to represent vascular endothelial cell damage due to abnormal lipid metabolism [36]. CAWS vasculitis initially develops from the adventitial side, and intimal thickening is not observed until the later stages of the inflammatory response. In Apo-E KO mice, however, the involvement of the medial elastic fibers and adventitia is hardly observed during the initial stage. From this perspective, the process of lesion formation greatly differs.
In the present model, vasculitis was induced in 100 % of the DBA/2 mice administered CAWS, and inflammatory responses were elicited in the initial stage following CAWS administration, which were so intense as to cause significant vascular lesions in a short period of time. CAWS vasculitis was not only limited to lesions in the aorta, but was also accompanied by advanced lesions of coronary artery origin, as well as in the aortic valve. The progression of CAWS vasculitis almost plateaus in about 4 weeks after administration, but in DBA/2 mice, lethal toxicity was observed from around 5 to 6 weeks. Furthermore, about 100 % died during the follow-up observation of a few weeks (Fig. 15.2). This trend is more significant in this strain than in other strains of high-CAWS-sensitivity mice, including C57Bl/6 and BALB/c mice. The cause of mortality in DBA/2 mice is difficult to explain solely on the basis of severe vasculitis, and since it takes several weeks of observation following the complete development of vasculitis until death, it is highly likely that sudden death was caused by the induction of fatal ventricular arrhythmia due to damage to the cardiac conduction system by remodeling, or complications with cardiac dysfunction due to aortic stenosis and regurgitation, or ischemic heart disease in DBA/2 mice with CAWS vasculitis. Furthermore, given that patients with acute heart failure or end-stage heart failure present with moist skin and cool extremities due to peripheral circulatory failure, as well as loss of appetite, it is possible that symptoms such as poor coat condition and weight loss, which appears to be due to decreased appetite, in DBA/2 mice might have resulted from heart disease complications. Taking these findings together, clarification of pathological conditions and cause of death in DBA/2 mice will require detailed studies, particularly one that focuses on the heart.
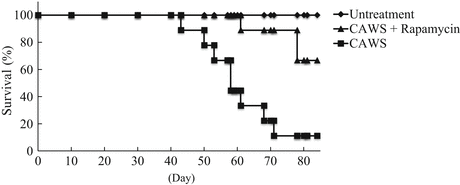
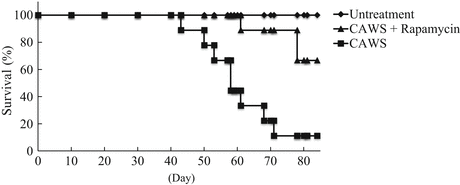
Fig. 15.2
Kaplan-Meier analysis of CAWS arteritis and efficacy of the therapeutic agent (rapamycin) in DBA/2 mice
CAWS-treated DBA/2 mice were administered with CAWS via peritoneal injections for five consecutive days. DBA/2 mice at 4 or 5 weeks of age were maintained in specific pathogen free (SPF) conditions and observed every day. Rapamycin (anti-inflammatory agent) was co-administered with CAWS
More detailed analysis of the pathological conditions of the present model will not only lead to the elucidation of vascular lesion formation mechanisms but also increase the utility of this strain as an excellent animal model to examine methods for treating cardiovascular diseases in preclinical stages.
15.3 Analysis of DBA/2 Mice as an Animal Model of CAWS Vasculitis with Left Ventricular Dysfunction
As described above, the murine model of CAWS vasculitis has contributed to the advancement of research as an animal model of KD. Despite this background, in humans, not all KD cases follow a simple clinical course of vasculitis alone, and some develop sequelae and complications such as coronary artery dilation and aneurysms, occasionally leading to death [6, 7]. Severe CAWS vasculitis is also induced in C57Bl/6 mice and BALB/c mice, but these other high-CAWS-sensitivity mice do not exhibit such severe lethal toxicity compared with DBA/2 mice [19]. Moreover, as mentioned above, the progression of CAWS vasculitis plateaus at 3–4 weeks after CAWS administration, whereas the lethal toxicity in DBA/2 mice was found appear after an observation period of several weeks. Taking these findings together, the lethal toxicity of CAWS vasculitis may not be primarily attributable to vasculitis itself. Rather, it is highly likely that valvular disease and ischemic heart disease occur in association with inflammatory changes in the aortic root, the opening of the coronary arteries, and around the aortic valve and the development of secondary cardiac dysfunction and complications during the interval period after the development of vasculitis leads to death. In this article, the complications of CAWS vasculitis in DBA/2 mice are considered, and the mechanisms underlying the severe lethal toxicity are discussed. In DBA/2 mice, we observed cardiomegaly as well as marked splenomegaly as organ changes following CAWS administration. In particular, cardiomegaly suggested cardiac complications associated with aortitis and coronary arteritis. Accordingly, in this article, we focus on structural and functional changes in the heart. In the first section, with regard to the lesions around the aorta, aortic valve, and myocardial tissue, we analyze morphological changes in inflammatory lesions and at the same time evaluate quantitatively the extent of cardiomegaly. In addition, we anatomically and histopathologically analyze CAWS vasculitis, with particular focus on the internal structure of the membrane tissue of the heart valve. Furthermore, in the second section, we analyze cardiac function in vivo by echocardiography, together with a comparative examination of B-type natriuretic peptide (BNP) expression, which is a useful diagnostic and prognostic marker of heart failure widely applied in clinical practice, between the group administered CAWS (hereafter, the CAWS group) and the control group [37].
As described above, aortic valve cusp thickening and significant deformation of the valve structure were observed in the CAWS group. In CAWS vasculitis, lesions did not simply manifest as vascular abnormality; rather, lesions also showed signs of potential severe morphological changes in the membrane tissue of the valve, mainly the aortic valve. In DBA/2 mice with CAWS vasculitis, we observed not only marked splenomegaly but also a significant heart weight increase with marked macroscopic cardiomegaly. In previous pathological analyses of tissue specimens, observations were performed using short-axis slices only; thus, it was difficult to obtain a full understanding of cardiovascular tissue. Accordingly, we prepared long-axis slices for histopathological analysis and comparison. Long-axis slices were prepared to be able to observe the aortic valve and ventricle simultaneously from the aorta through the apex. The observation of HE-stained long-axis heart tissue slices revealed that inflammatory lesions were not confined to the area around the aortic valve and the sinus of Valsalva, but also infiltrated into the interventricular septum directly below the aortic valve, as well as the myocardial tissue of part of the left and right ventricles. Furthermore, it was also revealed that, owing to the infiltration and accumulation of inflammatory cells (particularly valve cusp thickening from the left ventricle to the aortic valve and inflammatory lesions directly under the annulus of the aortic valve), severe stenosis developed, which morphologically extended from the left ventricular outflow tract (LVOT) to the opening of the aortic valve; this is a new finding. Moreover, the observation at the myocardial cell level revealed that the cross-sectional area of left ventricular myocardial cells increased by 1.14-fold in the CAWS group compared with that in the control group, and concentric hypertrophy was observed [37].
In the body, cardiovascular tissue exhibits circulatory dynamics with its lumen filled up with blood. Thus, histopathological analysis after the animal has been sacrificed does not allow for the evaluation of cardiac function, such as hemodynamics, or anatomical morphological changes in cardiovascular tissue in vivo. It is essential to observe the cardiovascular structure in the living organism and evaluate cardiac function in order to determine the causes of death in DBA/2 mice. In particular, in order to evaluate in vivo cardiac function immediately before their death, minimally invasive evaluation methods are needed. Ultrasound cardiography (UCG) is less invasive to the body, and as it enables real-time observation of organ morphology and function, it is essential in heart disease examination in clinical settings and has been used widely [38]. In a typical UCG examination, heart morphology (the form and movement of left ventricle, left atrium, aorta, mitral valve, and aortic valve) is observed with a probe placed on the chest wall. The most common type of heart tomographic image is a B-mode image. On the basis of this image, an M-mode echo image is obtained, which yields a one-dimensional view of the selected structure moving over time. Measurements of blood flow are possible by Doppler echocardiography. The main cardiac parameters obtained by UCG include the following: aortic diameter (AoD), left atrial dimension (LAD), left ventricular diameter (LVD) (LVDd = left ventricular end-diastolic diameter; LVDs = left ventricular end-systolic diameter), interventricular septal thickness (IVS) (IVSd = interventricular septal thickness at end diastole; IVSs = interventricular septal thickness at end systole), left ventricular posterior wall thickness (LVPW) (LVPWd = left ventricular posterior wall thickness at end diastole, LVPWs = left ventricular posterior wall thickness at end systole), left ventricular fractional shortening (LVFS) (%) ((LVDd – LVDs) / LVDd × 100), left ventricular ejection fraction (LVEF): estimated by several calculation methods), and inferior vena cava (IVC). Changes in cardiac function following CAWS administration were closely observed; in particular, cardiac function during the 6th to 7th weeks (i.e., immediately before death) was evaluated in detail by UCG. At the time of UCG evaluation, 10 mice each were prepared for the CAWS group and the control group; however, two of the CAWS mice died before examination, leaving eight available for the subsequent examination. However, two of the eight mice in the CAWS group died during UCG examination due to severe bradycardia leading to cardiac arrest. It was not possible to evaluate the cardiac function of these two mice immediately before their death; in these cases, the presence of severe heart disease was suggested [37]. The UCG of the six mice of the CAWS group, for which examination was feasible, revealed a diffuse, severe reduction of contractility in the left ventricle compared with the control group (Fig. 15.3). The segmental akinesia in the wall of left ventricle was not found. It was suggested that the influence of the ischemia did not cause infarction in CAWS arteritis. In the CAWS group, no clear changes in cardiac function were demonstrated for some time after the onset of vasculitis. However, after the development of vasculitis, the aortic valve outflow velocity tended to be higher than that in the control group, suggesting an increase in pressure load (pressure gap) due to the narrowing of the LVOT and aortic valve (Fig. 15.4). Above all, at the end stage, a significant increase in LVDd and a significant decrease in LVFS were observed, along with the thinning of the myocardial wall. It was revealed that DBA/2 mice with CAWS vasculitis develop eccentric hypertrophy associated with aortic regurgitation (AR), which is accompanied by significant left ventricular dilation and reduced left ventricular contractility immediately before death.
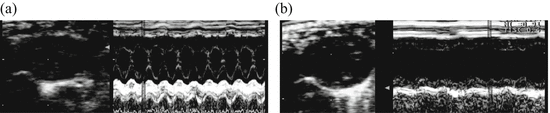
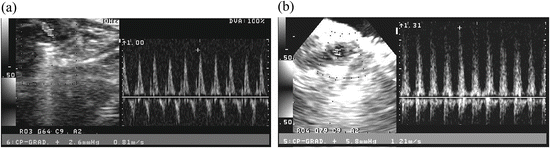
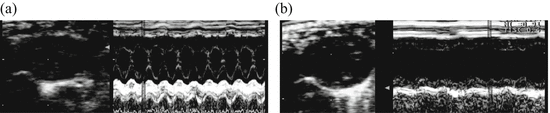
Fig. 15.3
Comparison of the cardiac function measured by echocardiography in untreated and CAWS treatment DBA/2 mice
CAWS treatment DBA/2 mice developed left ventricular dilution and dysfunction between 6 and 7 week after CAWS administration. (a) Untreated DBA/2 mouse, (b) The sixth week after CAWS administration
Left ventricular fractional shortening (LVFS) decreased from 71 % to 38 % (P < 0.01), and dilation of the left ventricular diastolic dimension (LVDd) was observed from 2.21 to 3.26 mm (P < 0.01) (b) n = 10 (untreated) and n = 6 (CAWS treatment)
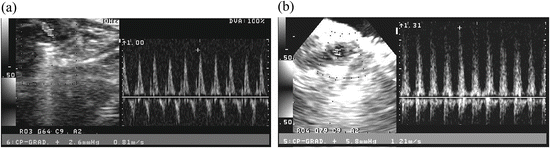
Fig. 15.4
Aortic valve and LVOT stenosis measured by echocardiography
The bloodstream increase (about 1.5 times) and pressure increase (more than two times) were found in the CAWS-treated DBA/2 mouse. (a) Untreated DBA/2 mouse, (b) The sixth week after CAWS administration
15.4 Assessment of Cardiac Function in DBA/2 Mice After the Onset of CAWS Vasculitis with B-Type Natriuretic Peptide (BNP)
Brain or B-type natriuretic peptide (BNP) and N-terminal (NT) proBNP are biomarkers of heart failure widely used in clinical settings in Japan [39]. BNP was named after the fact that it was originally discovered from porcine brain tissue in 1998 [40]. BNP and atrial natriuretic peptide (ANP), which had been identified before BNP [41], were both found to be secreted from myocardial cells in proportion to the severity of heart failure [42, 43]. Later, in 1990, C-type natriuretic peptide (CNP) was also identified [44]. Thus, among the members of the natriuretic peptide family, BNP is often referred to as the “B-type” natriuretic peptide. Most studies on the natriuretic peptide family have been conducted in Japan, from their discovery to application. Along with ANP, BNP has been considered as a myocardial stress marker [45], with its precursor proBNP produced in response to increased cardiac load in myocardial cells, which is then converted by a protease into active BNP (half-life, about 20 min) and inactive NT-proBNP (half-life, 60–120 min) [39]. In mice, there have been studies in which blood ANP concentration and systolic blood pressure were compared among different mouse strains. These showed no significant differences in blood ANP concentration between inbred strains of wild-type mice [46, 47], leading to the conclusion that it is a useful indicator of cardiac function. Measurements of BNP and NT-proBNP could thus serve as prognostic indicators of acute and chronic heart failure, with high evidence according to clinical practice guidelines [48]. The onset of heart failure leads to other well-known pathological conditions of impaired diastolic performance and reduced systolic performance. However, differential diagnosis of these two conditions cannot be made on the basis of BNP level. Combining BNP with physiological tests such as UCG, however, can lead to a more accurate diagnosis [49]. Murine BNP cDNA has been identified by Ogawa et al. [50], but anti-mouse BNP antibodies are not commercially available at present as a reagent. This made it difficult for us to measure BNP at the protein level. Accordingly, in this section, induction of BNP by CAWS administration was observed at the mRNA level by the reverse transcription PCR method using RNA primers, as previously reported [51]. In the CAWS group, a significant increase in BNP mRNA expression level was observed 6 weeks after CAWS administration (Fig. 15.5).
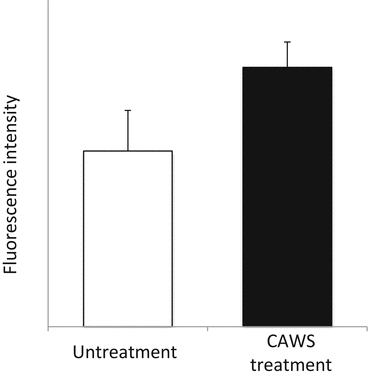
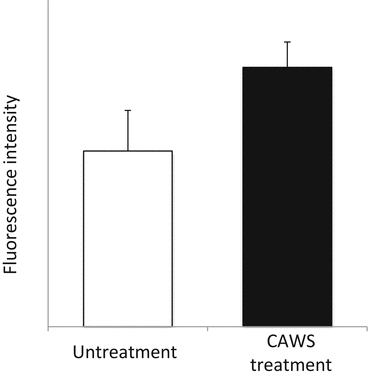
Fig. 15.5
mRNA expression of murine BNP in CAWS arteritis
The cDNA levels of murine BNP were examined by RT-PCR after 6 weeks CAWS administration
In this article, with regard to a sharp decrease in survival rate observed in the chronic phase of CAWS vasculitis in DBA/2 mice, we analyze mainly the effect of inflammatory lesions on the internal structure of the heart and cardiac function, with the aim of elucidating pathological conditions that may potentially lead to death [22, 37]. Valvular disease is a sequela of rheumatoid fever caused by group A hemolytic streptococcus infection and a representative inflammatory disease that could cause myocardial damage or cardiac dysfunction. This is believed to be due to an immunological cross-reaction between bacterium-derived component and autoantigen, which leads to the progression of valve tissue destruction [52]. Other acute diseases include viral myocarditis [53] and progressive destruction of the cardiac internal structure associated with acute pericarditis or infectious endocarditis. In these conditions, infections or inflammatory lesions greatly damage the internal structure and function of the heart and significantly affect patient prognosis [54]. In DBA/2 mice, although CAWS vasculitis is also accompanied by severe stenosis of coronary artery origin, previous studies showed no extensive infarct involving the left ventricle, scarred fibrous tissue, which is usually observed after myocardial infarction, inflammatory cell infiltration into inflamed myocardial tissue, or a valvular disease other than aortic valve disease. In addition, since ischemic heart disease by itself does not cause concentric hypertrophy with an increased myocardial cross-sectional area as a complication, CAWS vasculitis and accompanying left ventricular dysfunction might not have developed with ischemic heart disease as the direct underlying cause. In the present model, the major factor associated with concentric hypertrophy, which was observed in histopathological images, likely involves the narrowing of the LVOT directly under the aortic valve and the thickening and deformation of the aortic valve. These are developed as complications due to the progression of vascular lesions, which leads to the formation of inflammatory tissue around the aortic valve.
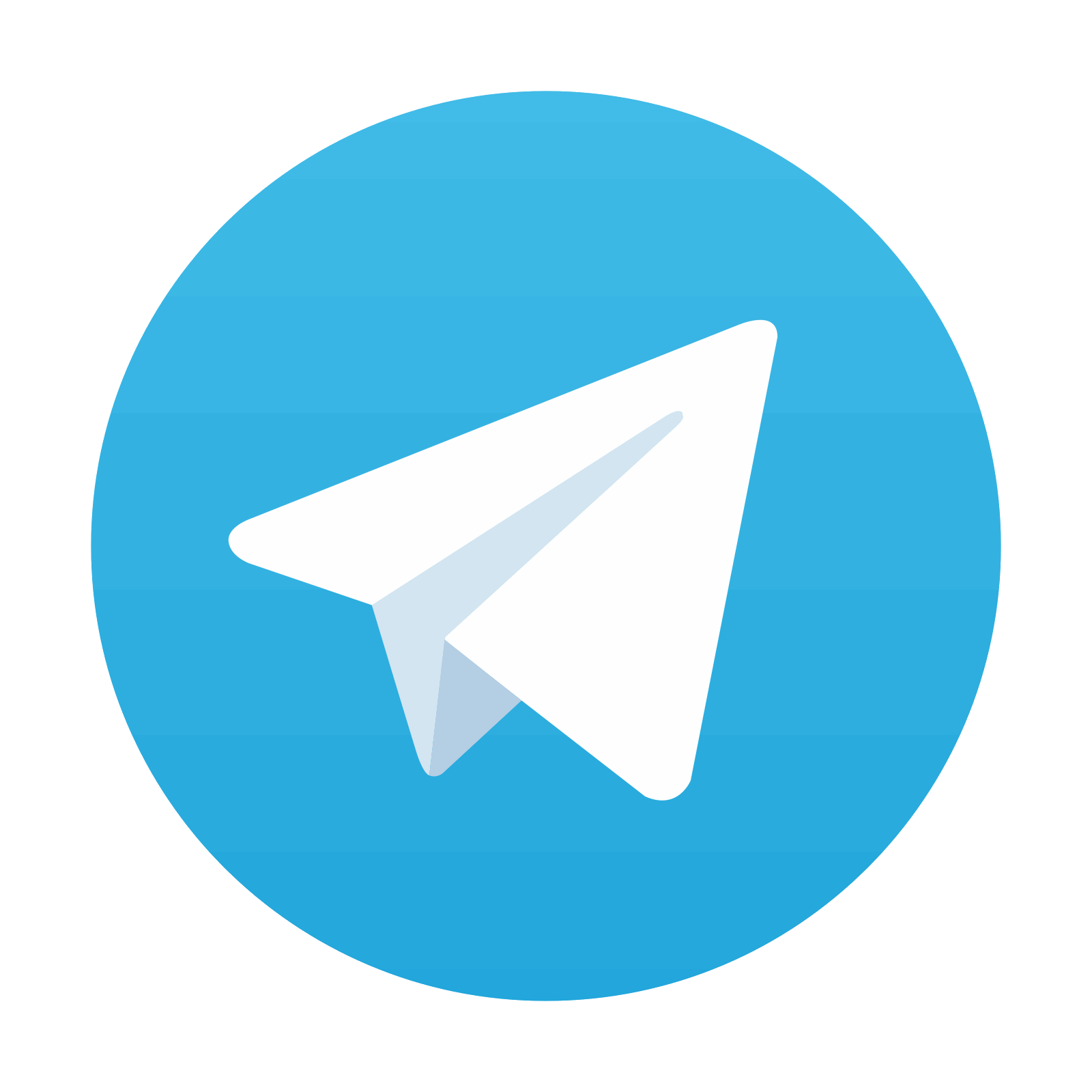
Stay updated, free articles. Join our Telegram channel

Full access? Get Clinical Tree
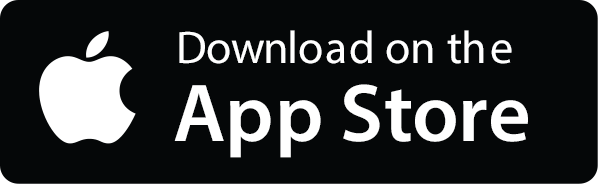
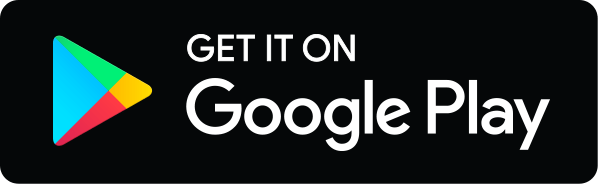