IUCN category
Number of speciesa
Response
Urgency
Extinct
34
Explore de-extinction; (cloning)
Not urgent, as long as governance and curation of frozen specimens is in place
Extinct in the wild
2
Implement ART; (cryobanking, hormone therapy, IVF)
Highest priority
Critically endangered
519
Implement ART; (cryobanking, hormone therapy, IVF)
Highest priority
Endangered
773
Implement ART; (cryobanking, hormone therapy, IVF)
High priority
Vulnerable
656
Develop ART procedures as contingency; (implement cryobanking)
Medium priority
Near threatened
390
Implement cryobanking
Low priority
Least concern
2,404
Implement cryobanking; select a few as model species for more advanced technologies
Low priority
Data deficient
1,633
Implement cryobanking
More information required
Total
6,411
2 Benefits of ART to Amphibian Conservation
Amphibian ART has gained considerable interest in the last decade but still lags behind technological developments for mammalian species. Although amphibian ART is a relatively new field of study, several recent advancements in protocol development have led to significant conservation achievements (Table 12.2) such as the production of endangered tadpoles from frozen-thawed sperm or the release of thousands of tadpoles produced by in vitro fertilization (IVF) (Kouba et al. 2009, 2013; Kouba and Vance 2009). When applied effectively, ART could result in increased breeding efficiency, reduced costs, halt loss of genetic diversity, reduce mate-pairing issues, and possibly even reject extinction as the only scenario for critically endangered species. Amphibian ART can achieve these outputs through the following mechanisms:
Table 12.2
Amphibian conservation achievements using assisted reproductive technologies
Application | Detail | References |
---|---|---|
Hormone-induced spawning in frogs and toads | Production of anurans in and out of season for research should reduce pressures on wild populations | |
Hormone-induced spawning and IVF in salamanders | Production of hellbenders (Cryptobranchus alleganiensis) for captive breeding program; production of tiger salamanders (Ambystoma tigrinum) as a model species for other ambystomids | Trudeau et al. (2012), Marcec et al. (unpublished) |
Use of IVF in captive breeding and release | 2,000 Wyoming Toads (Anaxyrus baxteri) produced by IVF; >10,000 boreal toads (Anaxyrus boreas boreas) produced by IVF; release of all tadpoles to the wild IVF performed in critically endangered Corroboree frog (Pseudophyrne corroboree) | |
Use of gamete transport between institutions, prior to successful fertilisation by IVF | Dusky gopher frog (Lithobates sevosa) sperm transported from Memphis Zoo to Omaha Henry Doorly Zoo, where IVF resulted in 200 eggs fertilised | |
Collection of sperm from wild males followed by cryopreservation, and IVF producing mixed wild-captive produced offspring | Sperm was collected from wild boreal toads (Anaxyrus boreas boreas), frozen in the field and used to fertilize eggs from hormone-induced females in captivity | Langhorne et al. (unpublished) |
Sexing of frogs using fecal or urinary steroids: NZ captive leiopelmatid frogs (urinary steroids); Australian captive bred Geocrinia juveniles (faecal steroids); N. American bufonids | Leiopelmatids are monoecious; sexing is required for mate pairing in captive breeding; Captive bred Geocrinia juveniles cannot be sexed visually; determination of captive bred juveniles prior to release to ensure even sex ratios at release sites |
2.1 Increased Efficiency of Captive Breeding and Release Programmes
Amphibian ART offers the potential to increase the efficiency of captive breeding by induced spawning or controlled release of gametes for IVF. Induced ovulation and spawning may remove the requirement for challenging behavioural and phermonal cues in recalcitrant species that may not otherwise breed readily in captivity, if at all (Table 12.2).
2.2 Reduce the Costs Associated with Captive Assurance Colonies by Limiting the Number of Live Animals Required to Sustain an Outbred Colony
By the combined use of small live populations with cryobanked gametes for breeding, heterozygosity and allelic diversity can be maintained with a fraction of the number of live animals. The savings per species are potentially enormous, with no reduction in effectiveness of the programme. Financial and logistical resources could be redirected to support more species and thereby contribute to the management of extinction risk.
2.3 Decrease the Rate of Genetic Diversity Loss in Captive and Small Wild Populations
The capacity of ART to improve genetic outcomes for species is under-recognised and under-utilised. Small wild populations, such as those managed on islands or remnant mainland populations, risk extinction from inbreeding depression (Frankham and Ralls 1998; Weeks et al. 2011). Captive populations in zoos and fenced sanctuaries risk loss of genetic fitness through selection for domestication (Williams and Hoffman 2009) that would reduce chances of later successful reintroduction to the wild. Storing genomes as gametes or embryos in the first or second generation after establishment of captive populations can prevent the loss of genetic diversity (Schad 2008). Long-term storage in gene banks offers many advantages to captive breeding programs (Table 12.3) and means that genetic diversity can be restored in small populations at any stage in the future. If diversity can be captured early in the process, the options for later restoring genetic fitness in wild populations are enhanced. Such genetic rescue can be very effective (Madsen et al. 1999; Westemeir et al. 1998).
Table 12.3
Justification for establishing Genetic Resource Banks for amphibians
Justification | Outcome for amphibian conservation |
---|---|
Reproductive failure | Incompatible breeding in amphibians could be overcome through the use of IVF |
Increased security | Provides some protection against disease outbreaks causing local extinctions to amphibian populations |
Unlimited space | Cryobanking offers a large amount of space to conserve diversity versus limited space and resources for live colonies |
Increased gene flow | Transportation of frozen gametes between zoos or wild populations (or between zoos and wild populations) has advantages over moving live amphibians |
Minimize introgression | Secures the integrity of a gene pool against the threat of hybridisation |
Extend generation times | The genetic lifespan is extended thereby reducing loss of alleles (genetic drift) |
Maximize genetic diversity | Storage of unrepresented founder amphibians, under-represented descendants and deceased animals |
Minimize inbreeding | Restoring germplasm to unrelated or more distantly related amphibians |
Manage effective population size | Equalize family size by manipulating age-specific fertility rates and sex ratios |
Minimize selection | Detailed pedigree analysis combined with GRBs can reduce genetic drift and increase genetic diversity for small assurance colonies |
Mutation | Extending generation lengths assists in decreasing the load of harmful mutations in small amphibian populations |
Safeguarding existing resources | Preservation of cell lines for future research and technological advances in ART |
Long-term benefits | Possibilities of restoring lost genes; discovering medicinal compounds for curing illnesses; pathogenic studies for disease resistance; nuclear transfer or parthenogenesis (gynogenesis and androgenesis) experiments |
Extinction risk | Reduce the risk of extinction |
De-extinction | Provide cells and genetic resource for the potential recovery of extinct populations and species with future technological advances |
2.4 Genetic Management Through the Control of Mate Pairing
This can be accomplished by selective breeding through induced spawning or IVF (Table 12.2). Genetic management of small populations requires the capacity to control the pairing of males and females; ART can overcome problems with mate choice where pairs show behavioural incompatibility, and thus slow the rate of loss of genetic diversity through more intensive management of captive animals (Schad 2008). For example, a female may produce thousands of eggs from hormone-induced spawning, which can be fertilized by sperm from multiple males during IVF.
2.5 Restoration of Extinct Species
A number of species that are extinct persist as frozen tissues (not cryopreserved) in various museums around the world. At one level, it is possible to argue that these lost species are not extinct because there is a high probability that the cell nuclei in the frozen specimens are still functional (in the same way that cells and gametes in cryostorage for human IVF labs, medical disease models or in agricultural industries are still functional and retrievable). Some consideration might be given to the possibility of retrieving at least some species as technology advances.
The ease of performing IVF in a taxon exhibiting external fertilization and development has resulted in the successful production of many amphibian species (Hollinger and Corton 1980; Browne et al. 1998; Edwards et al. 2004; Kouba et al. 2009, 2011). This includes the capacity to perform intra-cytoplasmic sperm injection (Kroll and Amaya 1996). The work relevant to reproductive technology has been concentrated in a relatively few laboratory species, especially of the genera Xenopus, Silurana and Lithobates (Rugh 1962; Lofts 1974; McKinnell 1978; Di Berardino 1997; Gurdon and Hopwood 2000; Schultz and Dawson 2003; Ogawa et al. 2011) and bufonids (Cabada 1975; Browne et al. 1998, 2001, 2002a, b, c, d, 2006a, b; Fitzsimmons et al. 2007). Yet there is a large body of published work on reproductive biology across many amphibian families (for comprehensive sources see Jamieson 2007; Ogielska 2009; Norris and Lopez 2011) that lay the foundations to develop captive breeding strategies for a wide range of species.
The impetus for research in amphibian developmental and reproductive biology has been due to the technical advantages of working with amphibian models in basic research, phylogeny and evolution. The focus therefore has not been on the applied aspects of amphibian reproduction or how this knowledge might assist conservation efforts. This area has developed slowly over the last two decades (Clulow et al. 1999; Kouba et al. 2009, 2013; Kouba and Vance 2009). Consequently, while some aspects of developmental and reproductive biology in amphibians are highly advanced, others relevant to their practical application and value in conservation biology are not. While reproductive endocrinology, nuclear transfer, and IVF are well developed in a few model laboratory species, other aspects such as genome storage via sperm, egg, embryo and somatic cells are well behind the advances made with other vertebrate taxa. In particular, very little has been studied in urodeles (salamanders and newts) or caecilians and the reader will note that most of the literature presented here is related to anurans (frogs and toads). Before exploring these topics in greater detail it is relevant to set the stage for understanding how the study of endocrinology has advanced our knowledge of amphibian reproductive mechanisms and allowed biologist to exploit the use of exogenous hormones for applied conservation.
3 Neuroendocrine Control of Reproduction
While amphibians share the main common reproductive neuropeptides, pituitary hormones and gonadal steroids with other vertebrates, very little is actually known about how the hypothalamic-pituitary-gonadal (HPG) axis is regulated, and therefore how it can be manipulated experimentally or for endangered species propagation. Here we review major aspects of the HPG axis that are directly relevant to induction of spawning for captive breeding. This review is pertinent given that obtaining gametes and fertilizing them (either through hormone-induced breeding or IVF) is central to the success of captive breeding programs where animals fail to breed naturally. Specific applications of injected hormones and agonists will be presented in later sections. The hypothalamic decapeptide gonadotropin-releasing hormone (GnRH) stimulates the synthesis and release of the gonadotropins from the anterior pituitary (Kim et al. 2011; Kah et al. 2007). The gonadotropins are luteinizing hormone (LH) and follicle stimulating hormone (FSH). These dimeric proteins are composed of a common alpha subunit and a differing beta subunit that confers specificity of binding to either the LH or FSH receptors, and thus also confers specificity of biological action at the level of the gonads. In vertebrates, natural ovulation in females and sperm release in males results from a surge release of LH (Fernandez and Ramos 2003; Trudeau 1997), so most ART research focuses on inducing LH release or stimulating LH receptors in the gonads. Little is known about the neuroendocrine regulation of FSH in amphibia.
3.1 Gonadotropin-Releasing Hormone
The neuropeptide GnRH exists in multiple forms across the vertebrate families (Kim et al. 2011; Kah et al. 2007). They are usually named for the species or animal group where they are first discovered. There are two GnRH forms in most amphibians that are the products of two different genes. These are mammalian GnRH (mGnRH; pGlu-His-Trp-Ser-Tyr-Gly-Leu-Arg-Pro-Gly-amide) and chicken GnRH-II (cGnRH-II; pGlu-His-Trp-Ser-His-Gly-Trp-Tyr-Pro-Gly-amide), also called GnRH1 and GnRH2, respectively. The hypophysiotropic neurons expressing mGnRH are restricted to the anterior preoptic area in the anuran brain and project to the median eminence (Collin et al. 1995). This indicates that mGnRH will be the predominant endogenous neuropeptide released into the portal blood vessels and delivered to the anterior pituitary to regulate gonadotropin synthesis and release in frogs. Neurons producing cGnRH-II are widely distributed throughout the frog brain (Collin et al. 1995). Thus, it could be speculated that cGnRH-II would exert central control over GnRH-regulated sexual behaviours rather than being the main regulator of gonadotropin release. It has recently been reported that mGnRH also regulates aspects of neurosteroid synthesis in the frog brain, implicating this GnRH form in the control of behaviour as well (Burel et al. 2013).
Elegant ligand–receptor interaction studies in several ranid species indicate high GnRH receptor (GnRH-R) selectivity for endogenous and synthetic analogs of GnRH (Wang et al. 2001; Seong et al. 2003). Relevant here is the observation that mGnRH exhibits high potency on the type 1 GnRH-R, the dominant receptor in the anterior pituitary of frogs. Therefore, efforts to evaluate the use of GnRH for spawning induction should focus on mammalian GnRH super-agonists because they will likely target the GnRH-R in the amphibian pituitary. To date the main GnRH agonists that are highly biologically active in anurans are des-Gly10– His(Bzl)6-GnRH-ethylamide and des-Gly10, d-Ala6, Pro9-GnRH-ethylamide (Kouba et al. 2009; Michael et al. 2004; Minucci et al. 1989; Waggener and Carroll 1998a, b; Browne et al. 2006a; Trudeau et al. 2010, 2013). The most promising to date is des-Gly10, d-Ala6, Pro9-GnRH-ethylamide because of high activity, longer half-life, availability, and low cost. It is a synthetically modified form of mGnRH. The modified alanine at position 6 is particularly important for increasing GnRH activity to stimulate LH release (Millar et al. 2004).
3.2 Control of Pituitary Gonadotropin Synthesis and Release
The distribution of gonadotrophs in the pars distalis (anterior) of the pituitary gland is similar to the situation in mammals (Gracia-Navarro and Licht 1987; Mizutani et al. 1994; Tanaka et al. 1990). Immunolocalization studies in several ranids indicate that the majority of gonadotrophs (~50–80 %) produce both LH and FSH, whereas the remainder produce only one of the gonadotropins. Very little is known about the factors controlling LH or FSH synthesis and release in amphibians. There are many studies exploring the role of a vast array of neuropeptides, neurotransmitters and sex steroids controlling gonadotroph function in fish (Peter et al. 1986; Trudeau 1997; Trudeau et al. 2000; Popesku et al. 2008; Zohar et al. 2010) and mammals (Barraclough et al. 1984; Herbison 1998; Smith and Clarke 2010). In marked contrast, most studies in frogs focus only on the stimulatory effects of GnRH on LH synthesis and release (Stamper and Licht 1990, 1993, 1994), which indicate a similar mode of action as found in other vertebrates. This includes upregulation of LH by GnRH self-priming (Porter and Licht 1985), a principle that has been demonstrated to enhance spermiation, ovulation and fertility in frogs (Silla 2011; Kouba et al. 2012a; Trudeau et al. 2013). Low doses of the neuropeptide GnRH can upregulate its own receptor, thereby enhancing the LH response to a specifically timed second injection (e.g., Trudeau et al. 2013). On the other hand, overstimulation by excessive GnRH treatments can have the opposite effect because GnRH receptors in the pituitary can be slowly down-regulated or desensitized (Pawson et al. 2008). Therefore, from a practical sense, the timing and frequency of GnRH treatments for induced breeding in a given species can have a significant impact on the outcome (i.e., success or failure).
Other data indicate that the pituitary hormone prolactin (PRL) can enhance GnRH-stimulated LH release from bullfrog pituitary cells in vitro; PRL cells are in close proximity to gonadotropin-producing cells, so it is probable the PRL is a paracrine factor (Oguchi et al. 1997). Prolactin can also act within the brain to enhance courtship behaviour in the male newts, Cynops pyrrhogaster (Toyoda et al. 2005). Therefore, environmental and hormonal factors modulating prolactin production could impact reproductive success in amphibia.
There are also few studies on the role of sex steroid feedback control of LH release in amphibians. Pavgi and Licht (1993) showed that estradiol (E2) has a direct inhibitory effect on FSH and LH secretion at the level of the bullfrog pituitary, indicating the existence of a negative feedback loop. In contrast, the androgen 5α-dihydrotestosterone (DHT) significantly elevated the GnRH-induced secretion of LH and FSH (Stamper and Licht 1994), which is evidence for positive feedback. On the other hand, in vivo studies indicate that both E2 and DHT suppress LH, perhaps indicative of decreased GnRH release in male leopard frogs (Tsai et al. 2005; Tsai and Jones 2005). Regardless, these data suggest the existence of both positive and negative feedback effects of sex steroids in frogs as reported in fish and mammals (Trudeau 1997; Trudeau et al. 2000; Zohar et al. 2010; Smith and Clarke 2010).
In leopard frogs it is known that estrogens and androgens can influence the number of forebrain neurons immunoreactive for tyrosine hydroxylase, a key enzyme in the catecholamine dopamine (DA) synthesis pathway (Chu and Wilczynski 2002; Wilczynski et al. 2003). However, the role that catecholamine neurons play in the steroidal feedback control of GnRH and LH is unknown. In goldfish it is clear that sex steroids modulate the synthesis of DA, and this is part of a negative feedback loop at the level of the brain (Trudeau 1997). Based on what is known in amphibians, we propose a model that can serve as a framework for future research on the hormonal control of spawning in amphibians (Fig. 12.1). While it is known that many other factors play a role in LH and FSH release in other vertebrates, they have been largely unexplored in the context of reproduction in amphibians.
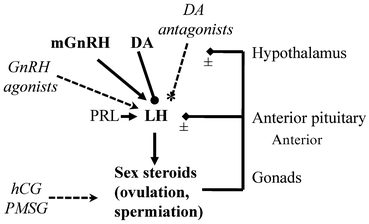
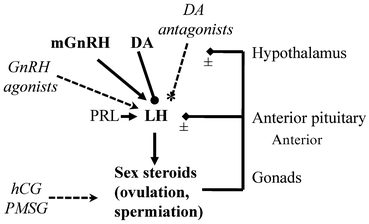
Fig. 12.1
Model for the neuroendocrine control of luteinizing hormone release in amphibians. The decapeptide gonadotropin-releasing hormone (GnRH) is produced in specific hypothalamic neurons in the hypothalamus. In amphibians the main endogenous hypophysiotropic form delivered to the anterior pituitary is mammalian GnRH (mGnRH). The GnRH is released into portal blood vessels at the median eminence. The GnRH is delivered to the anterior pituitary and acts on specific membrane-bound GnRH receptors on gonadotrophs to stimulate the production and release of luteinizing hormone (LH). The glycoprotein hormone LH is released from the pituitary into the general circulation and acts on specific LH receptors. For the ovary, LH receptors are found on theca and granulosa cells, and for the testes LH receptors are on the Leydig cells. The sex steroids (e.g., testosterone, estradiol, progesterone) are produced and released into the blood and act on specific receptors throughout the body to regulate numerous physiological processes. Shown here are the positive or negative feedback effects on LH release. Sex steroids can also act at the level of the hypothalamus to regulate GnRH and dopamine (DA), and sexual behaviors. The catecholamine DA is produced in specific hypothalamic neurons and is a potent inhibitor of LH release. It acts at the pituitary by binding to DA receptors. The pituitary hormone prolactin (PRL) can enhance GnRH-stimulated LH release. Also shown (italics, dashed lines) are the main hormone preparations currently in use for induced spawning in amphibia. Numerous natural and synthetic agonists of GnRH have been injected to stimulate LH release, leading to gamete release or spawning. They can be injected to stimulate gonadal LH receptors to induce sex steroid production, ovulation and spermiation. In amphibians, the mammalian gonadotropins human chorionic gonadotropin (hCG) and pregnant mare’s serum gonadotropin (PMSG) may have follicle stimulating hormone-like activities and stimulate FSH receptors on granulosa cells in the ovary to regulate follicular development or on FSH receptors on Sertoli cells in the testes to regulate spermatogenesis. Antagonists of DA have been used to potentiate GnRH action to super-stimulate LH release from the pituitary, which leads to amplexus and spawning. In this case, a GnRH agonist is co-administered with the DA antagonist. The DA antagonist blocks DA receptors, thus removing the inhibitory effects of DA on LH release (dashed line with asterisk). See text for further details
Similar to other vertebrates, seasonal profiles of amphibian hormones in relation to the timing of the breeding period indicate the importance of LH and FSH in controlling gonadal steroidogenesis and gonadal growth (Licht et al. 1983; Polzonetti-Magni et al. 1998; Itoh et al. 1990). Highly purified bullfrog LH and FSH exert season-dependent effects on ovarian production of E2 and progesterone in Pelophylax esculentus (Polzonetti-Magni et al. 1998). Interestingly, both LH and FSH were also shown to directly stimulate hepatic vitellogenin production in females (Polzonetti-Magni et al. 1998). Vitellogenin is the yolk glycoprotein under the stimulatory control of E2. Once produced in the liver, vitellogenin is released into the circulation, taken up by growing oocytes through receptor-mediated endocytosis, and serves to nourish developing embryos in oviparous vertebrates (Polzonetti-Magni et al. 2004).
3.3 The Role of the Catecholamine Dopamine
While it is known that GnRH and GnRH agonists activate recombinant GnRH receptors in vitro (Wang et al. 2001; Seong et al. 2003), and in some cases have been demonstrated to stimulate LH release, they may not effectively induce ovulation and spawning in frogs without in vivo co-treatments with other hormones or neuroactive agents (Browne et al. 2006a, b; Kouba et al. 2012a). This is highly suggestive of the existence of an inhibitory neuroendocrine mechanism controlling the surge release of LH. It has been known for more than two decades (Peter et al. 1986) that there are specific regions in the teleost forebrain that inhibits spawning by inhibition of LH release. In fish, a multitude of studies have clearly established that the main inhibitory substance is DA (Peter et al. 1986; Trudeau 1997; Dufour et al. 2005; Popesku et al. 2008). In marked contrast, central inhibitory control mechanisms have not been considered important in frog reproduction, even though there is convincing but sparse evidence. Electrolytic lesions in the hypothalamus and infundibular regions of the European frog Rana temporaria increased GnRH and LH release and advanced spawning times (Sotowska-Brochocka 1988; Sotowska-Brochocka and Licht 1992). Immunocytochemical visualization of DA neuronal fibres in the hypothalamus and median eminence of Pelophylax ridibundus and Pleurodeles waltl indicates that DA can be delivered to the amphibian pituitary (Gonzalez and Smeets 1991). In the túngara frog, Engystomops pustulosus, mapping of the catecholamine synthesis enzyme tyrosine hydroxylase in relation to the DA signaling protein DARPP32 indicated that these neuroendocrine regions contain DA and respond to DA (O’Connell et al. 2010). Moreover, the DA type 2 receptor is expressed in the frog pituitary (Nakano et al. 2010a, b). This neuroanatomical and biochemical evidence indicates that DA is potentially involved in the control of LH in amphibians.
More direct evidence for the involvement of DA also comes from studies of Rana temporaria (Sotowska-Brochocka et al. 1994). In vivo treatments with the DA type 2 receptor agonist bromocriptine, inhibits LH release and ovulation in Rana temporaria in some situations. In contrast, long-term treatment with a slow release implant containing the DA type 2 receptor antagonist metoclopramide (MET) induced ovulation in hibernating Rana temporaria (Sotowska-Brochocka et al. 1994). These data indicate that DA is an important inhibitor of LH release in frogs as it is in numerous fish species, birds and some mammals, including sheep and humans (Dufour et al. 2005). Browne et al. (2006a) explored the effects of combinations of hormones on spawning in Anaxyrus fowleri. In that study, they used the DA antagonist pimozide (PIM) and concluded that PIM may increase spawning in some situations and hormone combinations. Following this, Trudeau et al. (2010) proposed the use of combinations of GnRH agonists with DA receptor antagonists (PIM and MET) to induce spawning. It was reported that the combination of MET with the mammalian GnRH agonist des-Gly10, d-Ala6, Pro-GnRH-ethylamide was the most effective in Lithobates pipiens. Both PIM and MET cross the blood–brain barrier, and thus their sites of action can be both in the brain and pituitary and may be antagonist to endogenous DA action. It should be noted that PIM is not specific to DA receptors, and is known to act on adrenergic and serotoninergic receptors in addition to DA receptors (Bezchlibnyk-Butler and Jefferies 2005); therefore the use of PIM is not recommended.
The specific DA type 2 receptor antagonist domperidone (DOM) does not cross the blood–brain barrier, and acts at the level of the pituitary to potentiate GnRH action on LH release in goldfish (Omeljaniuk et al. 1987). In leopard frogs, the combination of des-Gly10, d-Ala6, Pro9-GnRH-ethylamide with either MET or DOM are equally effective at inducing spawning (Trudeau et al. 2013), so current evidence suggests that the major site of action of DA to potentiate LH release is the pituitary. Dopamine may have additional roles in anuran reproduction. Creighton et al. (2013) recently reported that activation of D2-like receptors by injection of a specific agonist has an inhibitory effect on vocalization in breeding male green tree frog, Hyla cinerea. It is therefore possible that DA acts in the brain to modulate sexual vocalizations and at the pituitary to control LH release to ensure that amplexus, oviposition, sperm release and fertilization are coordinated. Further experimentation is required to substantiate this hypothesis. A clearer understanding of the physiology, biochemistry, receptor signaling pathways and molecular mechanisms leading to the control of release of LH and FSH is critical for the development of specialized hormone treatments for applied conservation.
4 Collection of Gametes Following Injection with Exogenous Hormones
It is essential to understand the basic neuroendocrine control mechanisms and the site of hormone action in the body (Fig. 12.1). A wide array of hormones has been used in attempts to induce ovulation or sperm release in amphibians. These include synthetic GnRH agonists, purified pituitary gonadotropins (LH and FSH), human chorionic gonadotropin (hCG), pregnant mare serum gonadotropin (PMSG), and pituitary homogenates/extracts, alone or in combination with other agents. There are specific challenges with each hormone preparation, and lack of success with one method does not preclude success with another approach or a combination of therapies. Much remains to be discovered about the hormonal control of reproduction, and how to induce breeding in amphibians.
We recommend that new protocols applied to the induction of gamete release in any species for the first time should be initially tested on a subset of animals. This is particularly important if the protocols are being applied to rare or endangered animals that are critical to a captive breeding or reintroduction programme. Fatalities are extremely rare using the aforementioned hormones (<1 %), when administered properly (Kouba et al. 2012a). Potentially, these hormones could cause physiological upsets or even death if given too frequently (e.g., improper handling or multiple injections given within a 1 week span) or accidentally over-dosed due to human error. In the unlikely situation that an animal dies and it was a female, death may occur because of egg retention. In this scenario, the female would not have died as a direct result of the hormone therapy. Ovulation may have occurred but oviposition did not happen, so the retained eggs may lead to problems in such cases (Green et al. 2007).
It is clear that ART is having a positive impact on the reintroduction and restoration of wild amphibian populations. For example, to date, more than 100,000 endangered Wyoming toads have been produced collectively and released into the wild through the use of hormones for induced spawning. Similarly, over 250,000 threatened Puerto Rican crested toads and 50,000 boreal toads have been produced and released because of hormone therapy. In this context, it will be important to carefully monitor the health of amphibians produced via ART to ensure long-term success of re-established populations. The rest of this section will provide in detail information on several hormones used to induce egg maturation, ovulation, spermiation, and amplexus.
4.1 Use of GnRH Agonists for Spermiation and Ovulation
In amphibian literature, the natural decapeptide GnRH, and the many potent synthetic agonists, are often referred to incorrectly as luteinizing hormone-releasing hormone (LHRH). This is a misnomer because it does not specify the correct structure or source of the peptide. For consistency in this review we will refer to the peptide’s more appropriate classification as GnRH. There are several advantages of using GnRH agonists over other hormone therapies because it acts at the level of the pituitary (Fig. 12.1) to stimulate endogenous LH production and release. Although there are dozens of GnRH compounds available commercially, the most common analog used by the amphibian community to date is des-Gly10, d-Ala6, Pro9-GnRH-ethylamide (Bachem, catalog # H4070; also called des-Gly10, d-Ala6, LHRH ethylamide, Sigma-Aldrich, catalog #L4513). While limited comparative trials have been conducted in amphibians, most other GnRH agonists have not performed as well as des-Gly10, d-Ala6, Pro9-GnRH-ethylamide for the stimulation of spermiation and ovulation (Mann et al. 2010; Michael et al. 2004; Trudeau et al. 2010). This is likely because of reduced binding of the agonist to the amphibian GnRH receptor (Wang et al. 2001; Seong et al. 2003). Goncharov et al. (1989) tested this GnRH agonist on 39 different amphibian species and found several thousand-fold differences in apparent concentration potency needed to induce spermiation in males. This could be a true species difference in sensitivity and/or due to differences in the physiological state of the injected animals. It is advisable to consider GnRH plus a DA antagonist for less sensitive species so that endogenous LH release is maximized (Trudeau et al. 2010, 2012).
In males, GnRH has been shown to stimulate spermiation in a diverse array of anuran and some urodele species (Vellano et al. 1974; Trottier and Armstrong 1975; Waggener and Carroll 1998a, b; Goncharov et al. 1989; Minucci et al. 1989; Clulow et al. 1999; Obringer et al. 2000; Rowson et al. 2001; Kouba et al. 2012a; Browne et al. 2006a, b; Pozzi et al. 2006; Kouba et al. 2009; Mansour et al. 2010; Mann et al. 2010; Silla 2010; Byrne and Silla 2010; Silla and Roberts 2012). Moreover, injection of GnRH combined with the DA antagonist MET also stimulated spermiation in male leopard frogs (Trudeau et al. 2010, 2013). In female amphibians GnRH stimulates final egg maturation, ovulation, and spawning in a number of species as well (Waggener and Carroll 1998a; Whitaker 2001; Roth and Obringer 2003; Michael and Jones 2004; Michael et al. 2004; Kouba et al. 2009; Byrne and Silla 2010; Roth et al. 2010; Silla 2011)
4.2 Use of Chorionic Gonadotropins for Spermiation and Ovulation
Given the fundamental importance of gonadotropins to regulate gonadal function, numerous studies in amphibians have used these hormones to induce ovulation, spermiation or spawning, including hCG and PMSG (Clulow et al. 2012; Kouba et al. 2012a). These gonadotropins are produced outside the pituitary in placental tissues of select mammals, and may have both LH-like and FSH-like effects, depending on the species studied. These chorionic gonadotropins are relatively easy to obtain in large quantities from urine of pregnant women or mares and they have been used to partially mimic endogenous LH or FSH in numerous non-mammalian species. In the 1940s to 1960s the standard pregnancy test used ovulation or spermiation in amphibians to determine a positive or negative result (Bellerby 1934; Shapiro and Zwarenstein 1934; Galli-Mainini 1947; Gurdon and Hopwood 2000). After injection of human female urine into Xenopus laevis or Rhinella arenarum, the presence of eggs or production of sperm was monitored in the female or male anuran, respectively. If a woman was pregnant, there would be enough hCG in the urine to induce these responses in the amphibians. This method became known as the “Bufo test” for pregnancy. Injection of hCG does not have the same efficacy in amphibians as it does in mammals, therefore the effective dose for amphibians being nearly 2,000 times higher than that given to a mammal on a per weight basis (Kouba et al. 2009). Nevertheless, amongst the amphibians, a number of bufonids have been shown to produce gametes following administration of hCG (Clulow et al. 2012). In contrast, Licht (1995) found reduced sensitivity to hCG in Lithobates pipiens production of gametes and hypothesized this poor response was related to the low affinity of amphibian LH receptors for hCG in this species. Even though hCG shows reduced specificity, species-specific responses, and is required in high concentrations, it is widely used due to its commercial availability, standardized activity (International Units; IU) that can be adjusted on a per weight basis, and low side effects or reported health problems.
Injection of hCG has been shown to induce spermiation in a wide range of male amphibian species. It has proven effective in most bufonids tested to date including numerous species in the genus Anaxyrus and Rhinella (Iimori et al. 2005; Browne et al. 2006a; Kouba et al. 2009, 2012b). Moreover, an increasing number of amphibians have been reported to respond to hCG including those in the genus Lithobates and Rana (McKinnell et al. 1976; Easley et al. 1979; Kurian and Saidapur 1982; Rosemblit et al. 2006; Minucci et al. 1989; Uteshev et al. 2012; Subcommittee of amphibian standards 1996; Kouba et al. 2009, 2011), Leptodactylus species (Rosemblit et al. 2006), Xenopus species (Subcommittee of amphibian standards 1996) and Litoria species (Clulow et al. 1999). Administration of hCG can also induce ovulation in a number of other species, including Mixophyes fasciolatus (Clulow et al. 2012), Eleutherodactylus coqui (Michael et al. 2004), Engystomops pustulosus (Lynch et al. 2006), Ambystoma mexicanum (Mansour et al. 2011), several Bufonidae (Browne et al. 2006a, b; Kouba et al. 2009, 2013), and Xenopus laevis (Hollinger and Corton 1980).
Phylogenetic analysis of the gonadotropin beta subunits has shown that hCG and PMSG are protein products of genes only distantly related to amphibian LH beta and FSH beta (Fig. 12.2). This may help explain the wide range of hCG-sensitivities in amphibians. As illustrated in the phylogenetic analysis (Fig. 12.2), chorionic gonadotropins group most closely with mammalian LH beta subunits, being clearly separated from the amphibian LH beta subunit clade. The purification of sufficient amounts of endogenous amphibian LH and FSH is difficult, as is the production of recombinant gonadotropins that retain full biological activity. The gonadotropins are highly glycosylated, and these carbohydrate additions are critical for full biological activity. Therefore, strategies using homologous gonadotropins, which would be much more effective for amphibian ART, are unlikely to be available in the near future, although recombinant human LH and FSH have shown some promise in inducing spermiation in toads and were more effective than hCG-induced spermiation in A. arenarum (Pozzi et al. 2006). Hence, recombinant gonadotropins warrant more investigation for their usefulness to amphibian ART.
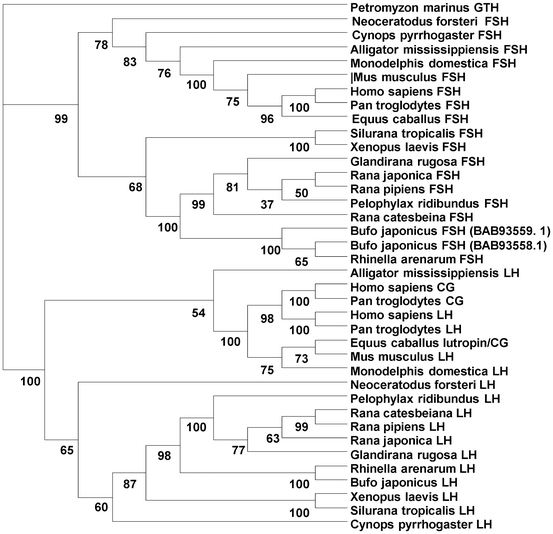
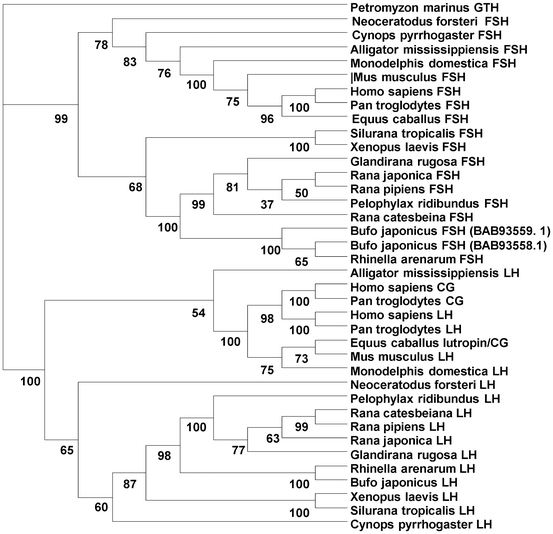
Fig. 12.2
Phylogenetic tree depicting the evolutionary relationships between the beta subunits of the vertebrate gonadotropins. The gonadotropins are composed of a common alpha subunit linked to a unique beta subunit which confers biological specificity. The main gonadotropins are luteinizing hormone (LH), follicle stimulating hormone (FSH), and the chorionic gonadotropins (CG). The gonadotropin (GTH) subunit from the lamprey Petromyzon mariunus was used as an outgroup. Shown is the consensus tree generated from DAMBE (Xia 2001; Xia and Xie 2001), with bootstrap support for individual nodes based on the aligned amino acid sequences. The Poisson-corrected distance and neighbor-joining method were used. See text for further details
In a number of cases, the use of a combination of a GnRH agonist and hCG have proven more effective at inducing spermiation or ovulation than either hormone alone, even when tested at higher individual doses (Browne et al. 2006a; Kouba et al. 2009, 2012a; Germano et al. 2011). These hormone combinations may be more effective because both the pituitary and gonad would be stimulated (Fig. 12.1). Additionally, the combination of hCG with PMSG was highly successful for stimulating ovulation in the barred frog Mixophyes fasciolatus (Clulow et al. 2012). In this case, the combinations of heterologous hormones were likely effective at stimulating both LH and FSH receptors in the ovaries (Fig. 12.1).
4.3 Use of Pituitary Homogenates for Inducing Spermiation or Ovulation
Pituitary homogenates are the excised pituitary gland from a sacrificed donor amphibian, with the gland then crushed in a suitable medium and subsequently administered to a recipient for purposes of breeding or obtaining gametes. Pituitary extracts go through a further crude purification step to concentrate the hormones and remove some of the cellular debris. Injections of pituitary homogenates stimulate ovulation and spawning in female amphibians and, to a lesser degree, spermiation in males (Subcommittee on amphibian standards 1996; Edwards et al. 2004). The use of pituitary homogenates was the first method for the collection and study of gametes from live animals. There are numerous disadvantages to using pituitary homogenates; therefore, their use should be avoided. First, a pituitary homogenate/extract may contain dangerous transmissible diseases that could be passed to the recipient. Given the global spread of pathogens, such as chytrid fungus and ranavirus, the passage of these diseases to endangered or threatened species must be avoided (especially if the future goal is reintroduction). Second, animals must be sacrificed to collect pituitaries. Researchers and conservationists must weigh the ethical and acceptable risk of this method. Third, the exact reproductive state and hormonal milieu of the donor animal(s) is typically not known and the active amount of gonadotropin available to the recipient can vary several thousand-fold. Fourth, the homogenate/extract is comprised of other cellular debris and/or pituitary hormones that can have adverse effects on the recipient. Most commercial supply companies have discontinued the sale of frog pituitaries possibly due to disease issues. However, if all other possible routes of hormone stimulation have been exhausted and failed to produce gametes, the ethical sacrifice of a common or invasive species should be considered as a mechanism to save a near extinct species. Although none of the authors endorse the use of pituitary homogenates in practice, there may be future circumstances where the technique may be employed, for example, harvesting pituitaries from an invasive species to use for induced reproduction in an endangered species.
5 In Vitro Fertilization and Short-Term Storage of Gametes
In vitro fertilization for amphibians has been performed for more than 60 years, predominantly for studies on early embryonic development or for commercial production of laboratory species. Since the detailed description of IVF in Xenopus (Wolf and Hedrick 1971), few IVF protocols have been applied to anurans outside of the common laboratory models. The development of IVF for amphibians is relatively simple compared to mammals because of the many advantages of external fertilization in water. Complex cell culture media and highly specific incubation conditions are all necessary to perform IVF in mammals due to the fact that natural fertilization is internal. For the most part, these complex protocols are not needed for amphibian IVF, which makes this technique highly amenable for captive amphibian breeding programs. Unfortunately, limited studies have been done for internally fertilizing urodeles (salamanders/newts) or for anuran species that do not have an aquatic life-stage (i.e., direct developers).
To conduct IVF for aquatic breeding amphibians, eggs are quickly removed from the water once spawned, placed into a dry Petri dish and mixed with spermatozoa for 5–10 min before flooding the dish with water (Kouba et al. 2009, 2012a). If eggs are stored in buffers containing electrolytes, they need to be rinsed in water several times before IVF and subsequent placement into the dry Petri dishes. Incubating the gametes in any buffer solution with an osmolality higher than 50 mOsmol per kg will inhibit fertilization (Edwards et al. 2004). It is likely that this inhibition to fertilization is due to inactivation of sperm motility at higher osmolalities (Browne et al. 1998; Kouba et al. 2003; Edwards et al. 2004). Typically, fertilization takes place with sperm concentrations ranging from 104 to 106 spermatozoa per mL (Wolf and Hedrick 1971; Browne et al. 1998; Edwards et al. 2004). Sperm concentrations for fertilization will likely vary between species and investigations should be undertaken to determine the best sperm to egg ratio for new species.
It was not until 1998 that Waggener and Carroll (1998a) conducted the first amphibian IVF in which both sperm and eggs were obtained from live males and females, even though the technique, using sperm collected post-mortem, had been employed in amphibians for more than 40 years prior to this report. These researchers found that both sperm and eggs could be collected from Lepidobatrachus species (L. laevis and L. llanensis) following an injection of a GnRH analog and when mixed together produced high rates of fertilization. This seminal paper demonstrated that ART for endangered amphibians was a real possibility. To date, IVF trials for threatened species have resulted in more than 2,000 Anaxyrus baxteri tadpoles produced and released to the wild (Browne et al. 2006a), over 10,000 Anaxyrus boreas boreas released to the wild (Kouba et al. 2013) and more than a 1,000 Lithobates sevosa produced by IVF but not released (Kouba et al. 2011). The endangered Pseudophryne corroboree frog has also been produced by IVF but all the embryos failed to develop and further studies are underway to improve these initial efforts (Byrne and Silla 2010). Methods for IVF have been developed in Ambystoma mexicanum (Mansour et al. 2011), Pelophylax lessonae (Uteshev et al. 2013), Xenopus laevis (Subcommittee on amphibian standards 1996), Pseudophryne guentheri (Silla 2011), several ranids (Shishova et al. 2011, 2013), Crinia georgiana (Dziminski et al. 2010), Limnodynastes tasmaniensis (Edwards et al. 2004), and Lepidobatrachus species (Waggener and Carroll 1998a). Toro and Michael (2004) were successfully able to produce offspring in a true direct developing frog (no aquatic life-stage), Eleutherodactylus coqui, using IVF. In addition, the first terrestrial salamander, Ambystoma tigrinum, was recently produced from IVF and hormone therapy for the collection of gametes (Marcec et al. unpublished; Fig. 12.3).
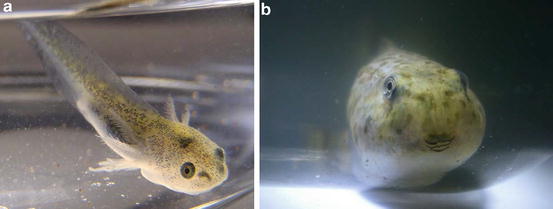
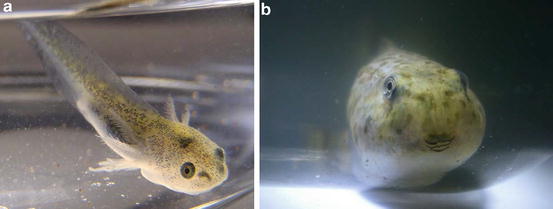
Fig. 12.3
Amphibian larvae produced through the use of hormone treatments and IVF at the Memphis Zoo and Mississippi State University. (a) Common tiger salamanders (Ambystoma tigrinum) have been produced by injecting hCG and a GnRH agonist to stimulate ovulation and spermatophore production, followed by IVF. (b) Critically endangered Dusky gopher frogs (Lithobates sevosa) created through a similar hormone therapy and IVF process, except that they were produced using frozen-thawed sperm held for months in cryostorage. Photographs: H. Bement
Gamete production in males versus females is often asynchronous when using ART; hence, the greatest challenge is typically storing gametes until the eggs or spermatozoa are obtained from the other sex. Amphibian sperm from a diversity of species has been shown to survive days or weeks at a time when stored at 4 °C (Browne et al. 2001; Kouba et al. 2009; Silla 2012) and do not generally appear to experience cold shock as in mammalian spermatozoa. The ability to store spermatozoa easily for extended periods in the refrigerator or ice slurry means that although the exact time of ovulation may be unpredictable, spermatozoa for fertilization can be readily available when eggs are eventually procured. Anuran eggs, on the other hand, are much more sensitive to short-term storage than spermatozoa. For the most part, once eggs are oviposited into water, the egg jelly quickly hydrates, resulting in structural changes that prevent sperm penetration after a short period of 30–60 min (Hollinger and Corton 1980; Elinson 1986; Olson and Chandler 1999). This can be circumvented to a limited degree by placing the eggs in a higher osmolality buffer solution (Browne et al. 2001; Edwards et al. 2004).
The short-term storage of gametes at temperatures above 0 °C can be an effective tool in ART where there is a need to transport sperm or eggs from one facility to another for IVF and genetic management. In the USA, cooled but unfrozen sperm of the endangered dusky gopher frog, Lithobates sevosa, were transferred from the Memphis Zoo in Tennessee to the Henry Doorly Zoo in Omaha, Nebraska where they were used successfully for IVF, sparing the need to transport live animals (Kouba et al. 2011, 2013). This represents the first time amphibian gametes have been shipped between institutions for the production of a critically endangered amphibian using hormone therapy and IVF technologies. Technological advancements for IVF, along with hormone therapy, are all valuable tools for amphibian conservation programs. In addition, the utility of stored gametes within a genetic resource bank depends on these previously developed techniques to successfully produce offspring for managed captive breeding programs.
6 Cryopreservation in Amphibian ART
6.1 Cryopreservation of the Male Germ-Line
Advances in amphibian cryobiology indicate that cryopreservation can play an important part in amphibian conservation (Table 12.2), and the establishment and operation of genome resource banks as a conservation action are as applicable to amphibians as for other vertebrate taxa (Holt et al. 1996; Bennett 2001; Holt 2001; Lermen et al. 2009; Rawson et al. 2011; Kouba et al. 2013). The creation of national amphibian genome resource banks (GRBs) that store somatic cells, tissues, gametes, embryos, and blood in a suspended state (typically in liquid nitrogen) has gathered momentum over the last several years (Kouba et al. 2013). Currently, GRBs have been established in Russia, the U.K., Australia, Germany, and the USA, housing a number of threatened and endangered species. While somatic cells and tissues are valuable for genomic, transcriptomic and proteomic studies, the real conservation value lies in the long-term preservation of gametes and embryos (Rawson et al. 2011). Cryopreservation of amphibian sperm has been shown to be a viable technology for an increasing number of species (Kouba et al. 2009, 2013; Browne and Figiel 2010) (see Table 12.2) since the pioneering work of Barton and Guttman (1972) and the achievement of the first fertilizations using frozen sperm (Browne et al. 1998). Typically, the use of GRB material requires the application of other advanced methods to produce live offspring.
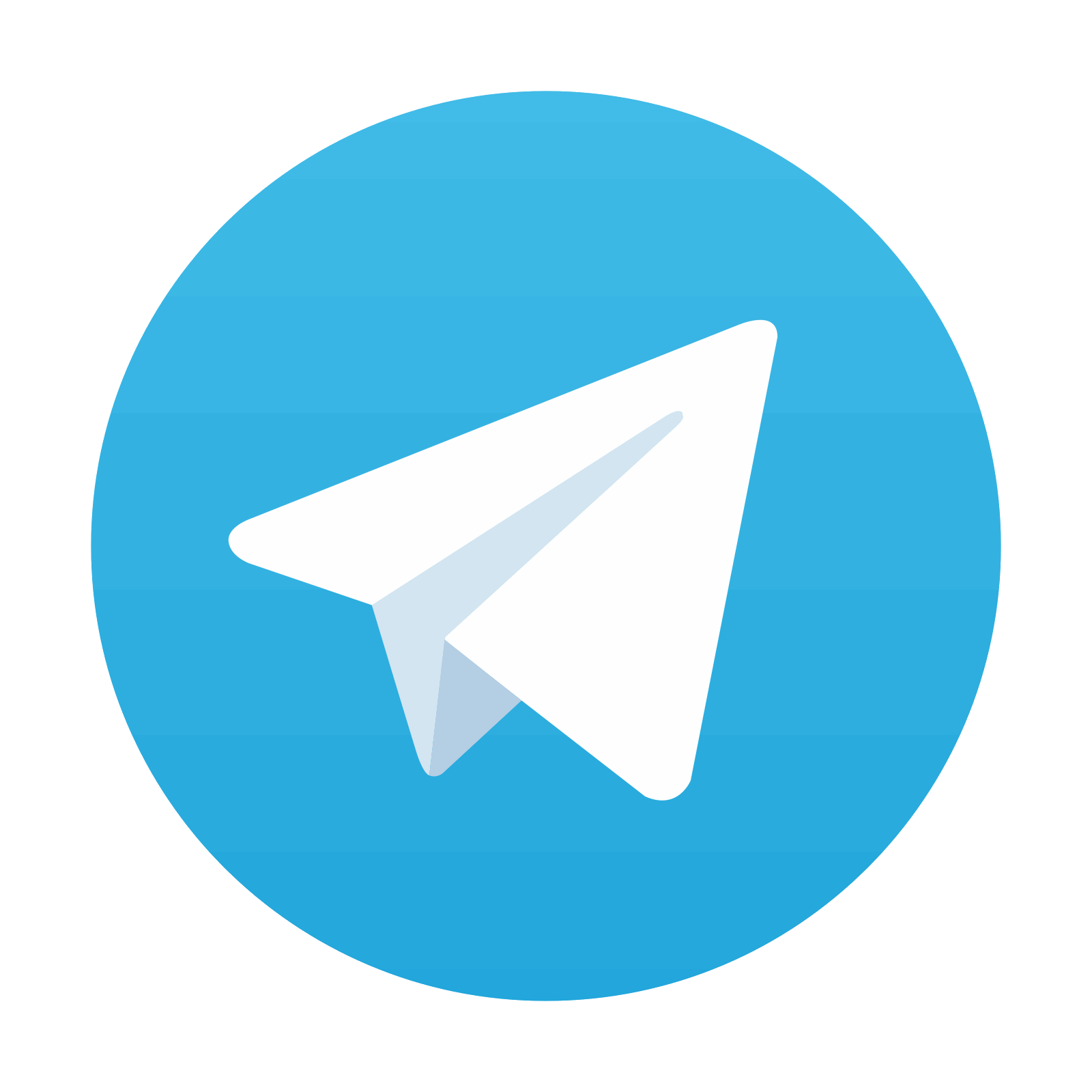
Stay updated, free articles. Join our Telegram channel

Full access? Get Clinical Tree
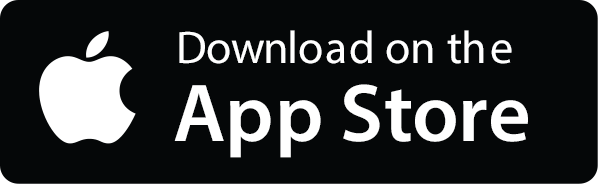
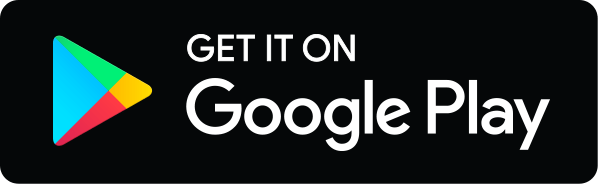