Indispensable
Dispensable
Conditionally indispensablea
Precursors of conditionally indispensable
Histidineb
Alanine
Arginine
Glutamine/glutamate, aspartate
Isoleucine
Aspartic acid
Cysteine
Methionine, serine
Leucine
Asparagine
Glutamine
Glutamic acid/ammonia
Lysine
Glutamic acid
Glycine
Serine, choline
Methionine
Serine
Proline
Glutamate
Phenylalanine
Tyrosine
Phenylalanine
Threonine
Tryptophan
Valine
For a long time, it has been assumed that all amino acids can enter the blood when absorbed by intestine epithelial cells. But more recently, it has been found that the small intestine can metabolize to a large extent some amino acids like dietary glutamate and most glutamine in addition to circulating glutamine derived from other tissues (Windmueller and Spaeth 1975). Glutamine (66 %), glutamate (98 %), and asparagine (99 %) are metabolized by the jejunal mucosa after uptake (Windmueller and Spaeth 1975, 1976). Recent in vivo studies have demonstrated extensive catabolism of many amino acids in the pig small intestine in the first pass (Yin et al. 2010). The biochemical bases for the metabolism of nonessential amino acids (NEAA) in pig enterocytes are now well established (Yin 2008). And metabolism of essential amino acids (EAA) is believed to play an important role in regulating the efficiency of utilization of dietary protein and amino acids (Le Floc’h et al. 1997).
Although the classification of the EAA and their assignment into a single category have been maintained in this report, the definition of NEAA has become blurred as more information on the intermediary metabolism and nutritional characteristics of these compounds has accumulated (Nutrition Board and Institute of Medicine 2005). Laidlaw and Kopple (1987) divided dispensable amino acids into two classes: truly dispensable and conditionally indispensable. Five of the amino acids in Table 11.1 are termed dispensable as they can be synthesized in the body from other amino acids.
11.2 The Function of Amino Acids
11.2.1 Metabolism of l-Amino Acid
Although the free amino acids present in the body fluids represent only a very small proportion of the body’s total mass of amino acids, they are very important for the nutritional and metabolic control of the body’s proteins.
The complex metabolism of amino acids can adapt to the physiological functions of intestinal tract and play important roles in the maintenance of intestinal structure and regulation of intestinal function.
Asparagine
l-Asparagine (ASN) is one of the NEAA, but it plays an important role in the metabolism. It can inhibit the function of monocarboxylate transporters (MCTs), which are sodium-independent transporters that mediate the update of aromatic acid and function as a net efflux pathway for aromatic amino acids in the basolateral epithelial cells (Kim et al. 2001) and regulate carbohydrate transport and metabolism in the body. Through the action of the asparagine synthetase, asparaginase-like protein 1 (ASRGL1) protein is involved in amino acid transport and metabolism (Fresquet et al. 2004; Al Sarraj et al. 2005; Overington et al. 2006). In addition, ASN is involved in the adjustment of energy production and conversion (Oppedisano et al. 2004; Dun et al. 2007).
In the pig intestine, ASN is a more stable amino acid in solution than glutamine but with similar structure. This amino acid can stimulate enterocyte cell proliferation (Rhoads et al. 1995). Asparagine synthetase is increased in cultured mouse spleen lymphocytes after stimulation by phytohemagglutinin, lignins, and lipopolysaccharide (LPS) (Hongo et al. 1989; Suzuki et al. 2002). Those studies suggest the possible link between the stimulated asparagine production and macrophage, lymphocyte activation.
Cysteine
Sulfur-containing amino acid (SAA) metabolism has been receiving increased attention because of the link to chronic diseases such as cardiovascular disease, Alzheimer’s disease, and diabetes. SAA have been regarded as involved in cardiovascular risk factors (O’Callaghan et al. 2005; Ozkan et al. 2002). In order to get a sulfur atom for subsequent sulfuration reactions, cysteine is first desulfurated by Nfs1 which transfers it onto a cysteine of multi-objective clustering selection operator section 3 (MOCS3), yielding a protein persulfide (Marelja et al. 2008).
l-Cysteine use by gut epithelial cells may play an important role for maintenance of glutathione synthesis and cellular redox function (Bauchart-Thevret et al. 2011). Glutathione synthesis is limited by l-cysteine availability, and there are many studies which have shown the potential for dietary l-cysteine intake to affect overall antioxidant status (Griffith 1999; Boebel and Baker 1983; Burgunder et al. 1989). Research on cysteine in the pig intestine has been often focused in relationship with N-acetylcysteine (NAC). This latter compound is the precursor for cysteine and glutathione production, therefore playing an important role in the protection of cells against oxidative stress (Wu et al. 2004). NAC reduces inflammation in the small intestine by regulating redox, epidermal growth factor (EGF), and Toll-like receptor-4 (TLR4) signaling (Hou et al. 2012a), and alleviates the mucosal damage and improves the absorptive function of the small intestine in LPS-challenged piglets (Hou et al. 2012b). When injury occurred in intestinal mucosa, the activities of antioxidant NAC, superoxide dismutase (SOD), catalase, and glutathione peroxidase (GPx) were enhanced. These enhancements might be related to the elimination of the superoxide radical and of H2O2, and are accompanied by a fall in glutathione-s-transferase (GST) and glutathione reductase (GR) activities (Cuzzocrea et al. 2000).
Histidine
l-Histidine is one of the EAA for many animals. l-histidine decarboxylase (HisDC) is the enzyme catalyzing the formation of histamine from l-histidine (Mamune-Sato et al. 1992). Recent report shows that histidine-rich glycoprotein (HRG) is an abundant plasma glycoprotein that has been shown to regulate a number of important biological processes, such as angiogenesis, cell adhesion and migration, fibrinolysis and coagulation, complement activation, immune complex clearance, and phagocytosis of apoptotic cells (Jones et al. 2005).
l-Histidine’s function can be related to histamine (HA) synthesis. Several reports suggest that HA is involved in the regulation of arousal state (Lin et al. 1990), locomotor activity (Clapham and Kilpatrick 1994), cardiovascular control (Imamura et al. 1996), water intake (Lecklin et al. 1998), and food intake (Leurs et al. 1998). Histamine is present in the mucosa of the gastrointestinal tract (Lorenz et al. 1973) and the intestine (Lee and Silverberg 1976; Linaker et al. 1981; Cooke et al. 1984; McCabe and Smith 1984). Histamine is the main mediator for inflammatory response; it can regulate immune function by stimulating receptors on target cells, these latter including hematopoietic progenitors, macrophages, platelets, dendritic cells, and T cells (Dy and Schneider 2004).
Tryptophan
l-Tryptophan is catabolized in seven steps to yield aminomuconate. Intermediates in this process are used for the synthesis of serotonin and kynurenine (Peters 1991). The serotonin 5-hydroxytryptamine (5-HT) neurotransmitter system contributes to various physiological and pathological conditions. 5-HT is the first neurotransmitter for which a developmental role was suspected (Nakamura and Hasegawa 2007).
l-Tryptophan is readily absorbed from the gastrointestinal tract (Yao et al. 2011). This amino acid is extensively bound to serum albumin. It is metabolized to serotonin and other metabolites and excreted in the urine. Pyridoxine and ascorbic acid appear to be related to the metabolism of tryptophan.
Tyrosine
l-Tyrosine is an NEAA, but it plays a key role in signaling transduction. Many signaling pathways do so by altering the phosphorylation state of tyrosine, serine, or threonine residues in target proteins (Pawson and Scott 1997). Tyrosine hydroxylase (TH) is the first enzyme in catecholamine biosynthesis, as well as being the rate-limiting enzyme in that process. TH requires tetrahydrobiopterin and uses iron as a cofactor in the 3,4-hydroxylation of tyrosine to produce dopa (Lewis et al. 1993; Flatmark 2000). Four isoforms of TH are expressed in the human brain and all of them possess the enzymatic activity (Lewis et al. 1993; Flatmark 2000).
l-Tyrosine can regulate absorption in small intestine (Musch et al. 1987). This amino acid is the substrate for the biosynthesis of the thyroid hormone thyroxine, the neurotransmitter dopamine, the adrenal hormones, and the pigment molecule melanin (Fitzpatrick 2003). A recent study has shown that acetaldehyde induces tyrosine phosphorylation and disrupts tight junction and adherent junction in human colonic mucosa. These latter effects can be prevented by EGF and glutamine (Basuroy et al. 2005).
Lysine
The function of l-lysine has been related to intestinal protection in pigs. l-Lysine may be a partial 5-HT 4 receptor antagonist and suppresses 5-HT 4 receptor-mediated intestinal pathologies (Smriga and Torii 2003). An increase in l-lysine intake might be a useful tool in treating stress-induced anxiety and 5-HT-related diarrhea-type intestinal dysfunctions (Smriga and Torii 2003). Recently the research on l-lysine has focused on l-lysine methylation and l-lysine-specific demethylase (Eliazer et al. 2011; DiTacchio et al. 2011). l-Lysine effect on the proteins of the ubiquitin–proteasome pathway has been described (Kensche et al. 2012; Van der Veen et al. 2011).
Methionine
l-Methionine is an EAA necessary for normal growth and development in mammals (Finkelstein 1990). l-Methionine is the precursor for cellular methylation reactions and for the synthesis of cysteine and can thus replace part of the cysteine requirement (Finkelstein et al. 1988; Finkelstein and Martin 1984; Mackay et al. 2012). Methionine salvage is important in humans for the recycling of sulfur that has to be assimilated in energy-consuming reactions (Pirkov et al. 2008; Albers 2009).
It is concluded that the transmethylation–transsulfuration sequence is the major pathway for l-methionine metabolism in the mammalian liver (Finkelstein 1990). l-Methionine is one of the EAA and it has very important functions in the intestine. SAA deficiency up-regulates intestinal l-methionine cycle activity and suppresses epithelial growth in neonatal pigs (Bauchart-Thevret et al. 2009). In proteins, one of the amino acids most easily oxidized is l-methionine, which is converted to l-methionine sulfoxide (Weissbach et al. 2002).
Phenylalanine
l-Phenylalanine and tyrosine constitute the two initial steps in the biosynthesis of dopamine, which, in turn, is the metabolic precursor of noradrenaline and adrenaline (Lou 1994). The extracellular l-phenylalanine concentration influences brain function in phenylalanine deficiency (PHD) by decreasing dopamine synthesis (Lou 1994). Phenylalanine hydroxylase (PheH) is an iron(II)-dependent enzyme that catalyzes the hydroxylation of aromatic amino acid l-phenylalanine to l-tyrosine (Zhang et al. 2011). Adrenaline, dopamine, and norepinephrine can be used as neurotransmitters and involved in the regulation of brain function.
l-Phenylalanine transport is depressed by l-tryptophan and l-methionine (Spencer and Samiy 1961). In the intestine, phenylalanine represents a competitive inhibitor involved in the regulation of the absorption of amino acids. There are also interactions which have been described between phenylalanine and the anionic amino acids, aspartate and glutamate (Philip et al. 2011). Phenylketonuria (PKU) is characterized by phenylalanine accumulation and progressive mental retardation. Phenylalanine assembly can form amyloid-like deposits, suggesting a new amyloidosis-like etiology for PKU (Adler-Abramovich et al. 2012).
Threonine
l-Threonine is also an EAA. This amino acid is the second or third limiting amino acid in swine or poultry diets behind the total SAA and lysine. So this amino acid can influence the balance of amino acids in vivo. This nutrient plays a critical role in the maintenance of intestinal mucosal integrity and barrier function (Mao et al. 2011).
Intestinal mucin synthesis is sensitive to dietary threonine supply, which suggests that the gut’s requirement for threonine may comprise a significant proportion of the whole body requirement (Nichols and Bertolo 2008). l-Threonine is of great importance for the maintenance of intestinal health. It is an EAA that is abundantly present in glycoproteins produced by the intestine (Sophie RD et al. 2007). The high requirement of the gut for l-threonine has often been ascribed to the synthesis of mucins, which are secreted glycoproteins protecting the intestinal epithelium from injury (Mao et al. 2011; Remond et al. 2009). This requirement could be even greater during intestinal inflammation, when mucin synthesis is enhanced and there is no oxidation of l-threonine by enterocytes (Remond et al. 2009). Because mucin proteins are little digested and reused, intestinal mucin secretion represents a net loss of l-threonine for the body (McCabe and Smith 1984).
Alanine
Among the 20 amino acids, l-alanine is the principal amino acid released by muscle, in accordance with the fact that alanine is the principal amino acid extracted by liver for gluconeogenesis (Felig et al. 1970). l-Alanine plays a major role in the transfer of nitrogen from peripheral tissue to the liver. This amino acid is involved in the metabolism of amino acids and derivatives (Yang et al. 2002), vitamins and cofactors (Marelja et al. 2008), nucleotides (Lee et al. 1995; Tamaki et al. 1990), l-phenylalanine and l-tyrosine catabolism (Wu 1998), (Han et al. 2009; Rossi et al. 2004), l-tryptophan catabolism (Perry et al. 1995), and glyoxylate metabolism (Rodionov et al. 2010). l-alanine is protective against the buildup of toxic substances that are released into muscle cells when muscle proteins are broken down quickly to meet energy needs, as it happens with aerobic exercise (Chang and Goldberg 1978). l-Alanine strengthens the immune system, boosts muscular anaerobic endurance and muscular strength, and increases aerobic endurance (Chang and Goldberg 1978).
Arginine
l-Arginine functions in the body are related to its utilization as a free amino acid, as a component of most proteins, and as the substrate for several nonprotein/nitrogen-containing compounds (Nieves and Langkamp-Henken 2002). l-Arginine participates in multiple pathways with important nutritional and physiological functions, including the synthesis of protein, nitric oxide (NO), creatine, proline, glutamate, and polyamines as well as the secretion of hormones (Tan et al. 2009a, b, 2011; He et al. 2009; Li et al. 2007; Yao et al. 2008). l-Arginine which enhances the immune system stimulates cell division, increases wound healing and hormone secretion, and regulates the vascular tone and many other various physiological processes (Nieves and Langkamp-Henken 2002). l-Arginine is the main substance of the urea cycle, which yields urea as the major form in which excess nitrogen is excreted from the human body (Brusilow and Horwich 2001). l-Arginine also is the main substance involved in the metabolism of polyamines (Moinard et al. 2005).
NO, a multifunctional second messenger, is implicated in numerous physiological functions in mammals that range from immune response and potentiation of synaptic transmission to dilation of blood vessels and muscle relaxation (Pacher et al. 2007). NO is also a regulator of intestinal electrolyte transport (MacNaughton 1993), and it has a dual excitatory and inhibitory effect on intestinal motility (Holzer et al. 1997; Tan et al. 2012a, b; Kong et al. 2012; Liu et al. 2012; Wu et al. 2012). l-Arginine is the only substrate for the synthesis of NO. As the precursor of NO, arginine possesses many functions related to effects on the immune system.
Aspartate
Aspartate is an NEAA in mammals, being produced from oxaloacetate by transamination. Oxaloacetate is one of several 2-oxoacids that function efficiently in the computational formalization of cognitive binary logic (CCBL1)-mediated transamination (Han and Li 2004) and oxaloacetate is an important substance involved in gluconeogenesis. The active form of the enzyme asparagine synthase (ASNS) is a dimer which can catalyze the reaction of aspartate, glutamine, and ATP to form asparagine, glutamate, AMP, and pyrophosphate (Van Heeke and Schuster 1989). Moreover, aspartate donates one nitrogen atom in the biosynthesis of inosine, the precursor to the purine bases (Chen et al. 2005). The glutamate N-methyl-d-aspartate receptor (NMDAR) plays a critical role in learning and memory (Burgdorf et al. 2011). Aspartate also stimulates NMDAR, though not as strongly as the amino acid neurotransmitter glutamate does (Chen et al. 2005). In the intestine, aspartate can affect the absorption of nutrients and, as the dietary supplement, can modify the intestinal ecosystem.
Citrulline
Citrulline is a key intermediate in the urea cycle, the pathway by which mammals excrete ammonia. Citrulline is made from ornithine and carbamoyl phosphate in one of the central reactions in the urea cycle (Rabier and Kamoun 1995). In cells, NO was synthesized from citrulline (via arginine) as well as from arginine, indicating the operation of the citrulline–NO cycle involved in arginine recycling (Mori 2007). Although citrulline is not coded for by DNA directly, several proteins are known to contain citrulline as a result of a posttranslational modification. Circulating citrulline is emerging as an innovating biomarker candidate for assessment of intestinal function (Crenn et al. 2011). Citrulline is a precursor for the production of arginine by the kidney (Crenn et al. 2011).
Glutamine, as arginine is a precursor of ornithine, which can be converted to citrulline by the intestine (van de Poll et al. 2007). Hepatic citrulline uptake limits the amount of gut-derived citrulline reaching the kidney and this may have implications for interventions aimed at increasing systemic arginine concentrations (van de Poll et al. 2007).
Glutamate
Monosodium glutamate is the crystalline salt of glutamate often used as a flavor enhancer. In neuroscience, glutamate is the most abundant excitatory neurotransmitter that plays a key role in long-term potentiation as well as in learning and memory (Burgdorf et al. 2011; McEntee and Crook 1993). Glutamate is a key molecule in cellular metabolism and can be involved in the synthesis of some amino acids.
Glutamine
The amino acid glutamine plays a central role in nitrogen transport within the body and is a fuel for rapidly dividing cells, such as in the gut and the immune system (Novak et al. 2002). It has been reported that glutamine has the ability to regulate gene expression in a number of physiological processes (Curi et al. 2007). Glutamine is also involved in amino acid synthesis and interconversion (transamination). These reactions mediate the synthesis of aspartate, asparagine, glutamate, and glutamine from ammonia or the intermediate of glycolysis, and allow the utilization of the carbon atoms from these four amino acids for glucose synthesis under fasting conditions.
Ko et al. (1993) show that l-glutamine, when added in amounts greater than required to prevent apoptosis, is essential for the EGF stimulation of cell proliferation as well as DNA, RNA, and protein synthesis in IEC-6 cells. This in vitro study further extends our knowledge on the important role of GLN in intestinal cell growth and function, and is consistent with a large number of in vivo studies demonstrating gut-trophic effects of this amino acid (Burke et al. 1989; Souba et al. 1992).
Ornithine
Ornithine has several common characteristics with citrulline. Both of them play a key role in the urea cycle. Ornithine is also not coded by DNA, and, in that sense, is not involved in protein synthesis (Weber and Miller 1981). Ornithine can realize the reversible transfer of an acetyl-group to an acidic (glutamate) amino acid by employing a common mechanism involving an acetyl-enzyme intermediate, but using different side chain binding modes, which is catalyzed by ornithine acetyl-transferases (Iqbal et al. 2011).
Ornithine decarboxylase (ODC) is the first enzyme of the polyamine biosynthetic pathway. ODC is induced by androgens in the mouse kidney. ODC in the small intestine is stimulated three- to tenfold by refeeding or administration of insulin (Maudsley et al. 1976). The upregulation of proglucagon and ODC gene expression may be the mechanism by which short-chain fatty acids (SCFAs) facilitate intestinal adaptation (Tappenden et al. 1996). Systemic administration of SCFAs has been shown to facilitate adaptation to small bowel resection (Tappenden et al. 1996). It has been shown that ODC activity in macroscopically normal tissue of patients with tumors of stomach and large intestine increased as the disease progressed. In addition, there is an inverse relationship between ODC activity in adenocarcinomas and differentiation (Berdinskikh et al. 1991). Thus in the intestine, ODC is indeed an important regulator and plays a role in cell signaling.
11.3 Amino Acids, Gene Expression, and Cell Signaling
Many studies have shown that glutamine modulates expression of genes that are crucial for intestinal metabolism and function. Dietary glutamine supplementation increased intestinal expression (120–124 %) of genes that are necessary for cell growth and removal of oxidants while reducing (34–75 %) expression of genes that promote oxidative stress and immune activation (Wang et al. 2008). Previous studies have shown that glutamine regulates the mitogen-activated protein kinase (MAPK) activation and c-Jun signaling in enterocytes (Rhoads 1999). Son et al. (2005) found that histidine significantly inhibited both hydrogen peroxide and tumor necrosis factor-alpha (TNF-α) induced interleukin-8 (IL-8) secretion as well as mRNA expression in intestinal epithelial-like Caco-2 cells and HT-29 cells. Moreover, other amino acids also contribute to regulate the intestinal metabolism since treatment of human intestinal cell line with branched-chain amino acids (leucine, isoleucine, or valine) down regulated the expression of the sterol regulatory element-binding protein-2 (SREBP-2), a transcription factor involved in cholesterol metabolism (Chen and Reimer 2009). Finally, amino acids such as branched-chain amino acids, glycine, lysine, threonine, and cysteine may also play important regulatory roles in the intestine, particularly in improving the immune function (Li et al. 2007; Calder 2006; Burrin and Stoll 2007; Newsholme et al. 2011; Wang et al. 2009).
It seems that the functions, in which amino acids are implicated as gene expression regulators, involve the activation of different signaling pathways and transcription factors. In fact, the regulation to gene expression is editing the structure of RNA, including deadenylation-dependent mRNA decay, microRNA-mediated RNA cleavage, regulation of mRNA stability, and tRNA aminoacylation. The two main processes by which amino acids regulate gene expression are control of tRNA aminoacylation and regulation of mRNA through signaling pathway.
11.3.1 Amino Acids Regulate Aminoacylation of tRNA
tRNAs are directly involved in the translation process of gene expression. In that way, they can influence the gene expression. tRNA synthetases catalyze the ligation of tRNAs to their cognate amino acids in an ATP-dependent manner. The reaction is shown in Fig. 11.1. The rapid hydrolysis of pyrophosphate makes these reactions essentially irreversible under physiological conditions (Fersht and Kaethner 1976a). Specificity of the tRNA charging reactions is achieved both by specific recognition of amino acid and tRNA substrates, by the synthetase, and by an editing process in which incorrect aminoacyladenylate molecules (e.g., valyladenylate associated with isoleucyl tRNA synthetase) are hydrolyzed rather than conjugated to tRNAs in the second step of the reaction (Baldwin and Berg 1966a, b; Fersht and Kaethner 1976b). The tRNA synthetases can be divided into two structural classes based on conserved amino acid sequence features (Burbaum and Schimmel 1991).
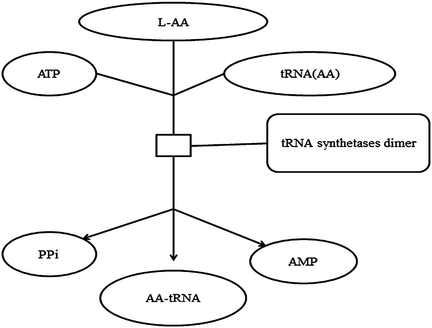
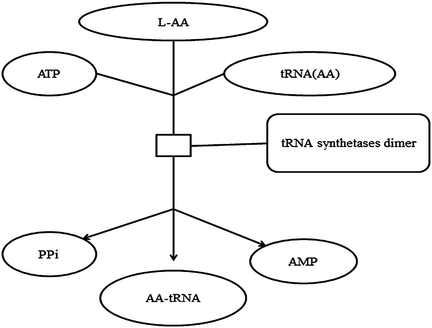
Fig. 11.1
L-AA tRNA aminoacylation. The reaction proceeds in two steps. First, amino acid and ATP form an aminoacyladenylate molecule, releasing pyrophosphate. The aminoacyladenylate remains associated with the synthetase enzyme where in the second step it reacts with tRNA to form aminoacyl tRNA and AMP
A number of tRNA synthetases are known to have functions distinct from tRNA charging (Park et al. 2005). Additionally, mutations in several of the tRNA synthetases, often affecting protein domains that are dispensable in vitro for aminoacyl tRNA synthesis, are associated with a diverse array of neurological and other diseases (Park et al. 2005, 2008; Antonellis and Green 2008). These findings raise interest into the role of these enzymes in human development and disease.
11.3.2 Amino Acids Regulate Gene Expression Through Cell Signaling Pathway
The best-characterized example of amino acid-induced regulation of a signal transduction pathway is one involving a protein kinase referred to as the mammalian target of rapamycin (mTOR), which acts to modulate the function of proteins engaged in both global mRNA translation and the selection of specific mRNAs for translation (Kimball and Jefferson 2004).
mTOR in most organisms has evolved to be able to make a transition between anabolic and catabolic states. This would allow these organisms to survive and grow in environments in which nutrient availability is variable (Laplante and Sabatini 2012). There are two mTOR complexes and both mTOR complexes are large. mTORC1 is known to have six and mTORC2 seven known protein components. They share the catalytic mTOR subunit, and also mLST8 (also known as GbL) (Jacinto et al. 2006; Kim et al. 2003), DEP domain containing mTOR-interacting protein (DEPTOR) (Peterson et al. 2009), and the Tti1/Tel2 complex (Kaizuka et al. 2010). In contrast, regulatory-associated protein of mammalian target of rapamycin (raptor) (Hara et al. 2002; Kim et al. 2002) and proline-rich Akt substrate 40 kDa (PRAS40) (Sancak et al. 2007; Thedieck et al. 2007; Wang et al. 2007) are specific to mTORC1, whereas rapamycin-insensitive companion of Mtor (rictor) (Jacinto et al. 2004; Sarbassov et al. 2004), mammalian stress-activated map kinase-interacting protein 1 (mSin1) (Jacinto et al. 2006; Frias et al. 2006), and protein observed with rictor 1 and 2 (protor1/2) (Thedieck et al. 2007; Pearce et al. 2007, 2011) are only part of mTORC2. Figure 11.2 describes the known molecular functions of amino acid-induced regulation of the mTOR complex.
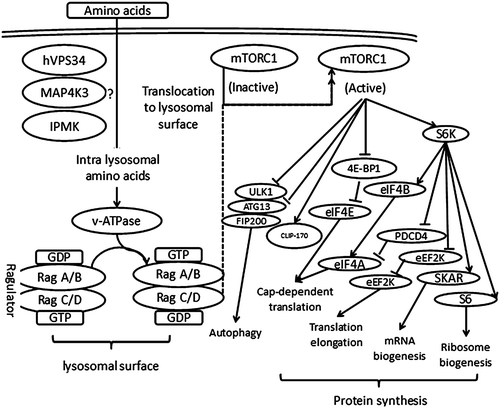
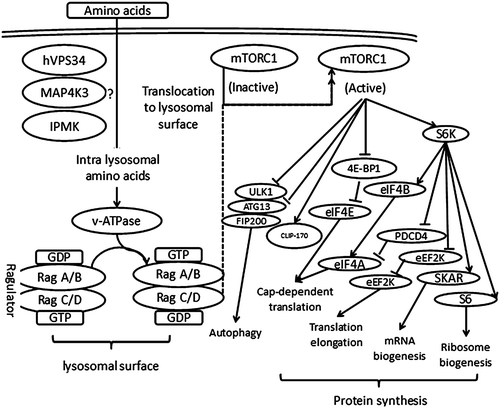
Fig. 11.2
The molecular mechanisms involved in the amino acid regulation of gene expression through the mTORC1 signaling pathway
Amino acids are the nutrition signal which can induce activated mTORC1. When activated, mTORC1 promotes protein synthesis mainly by phosphorylating the kinase p70 S6 ribosomal kinase (S6K) and the translation regulator of the eIF4E-binding proteins (4E-BP1) (Ma and Blenis 2009). This complex also induces lipid biogenesis by activating SREBP1 and PPARg transcription factors (Laplante and Sabatini 2009) and inhibits catabolism by blocking autophagy through the phosphorylation of the ULK1–Atg13–FIP200 complex (Ganley et al. 2009; Hosokawa et al. 2009).
Besides the mTOR pathway, many amino acids show their own way to reflect the cell signaling. In that sense, asparagine and glutamine are good examples. Asparagine starvation by asparaginase (ASNase) enhances phosphorylation of the eukaryotic initiation factor 2 (eIF2) by general control non-depressible 2 (GCN2) kinase, leading to reduced global mRNA translation rates. This conserves energy and allows cells time to reprogram stress-related gene expression to alleviate cell injury (Bunpo et al. 2010). Asparaginase depletes circulating asparagine and glutamine, activating amino acid deprivation responses (AADR) such as phosphorylation of eukaryotic initiation factor 2 (p-eIF2) leading to increased mRNA levels of asparagine synthetase and CCAAT/enhancer-binding protein-homologous protein (CHOP) and decreased mTORC1 signaling (Bunpo et al. 2009). NAC inhibits activation of c-Jun N-terminal kinase, p38 MAP kinase, and redox-sensitive activating protein-1 and nuclear factor kappa B transcription factor activities regulating expression of numerous genes (Zafarullah et al. 2003).
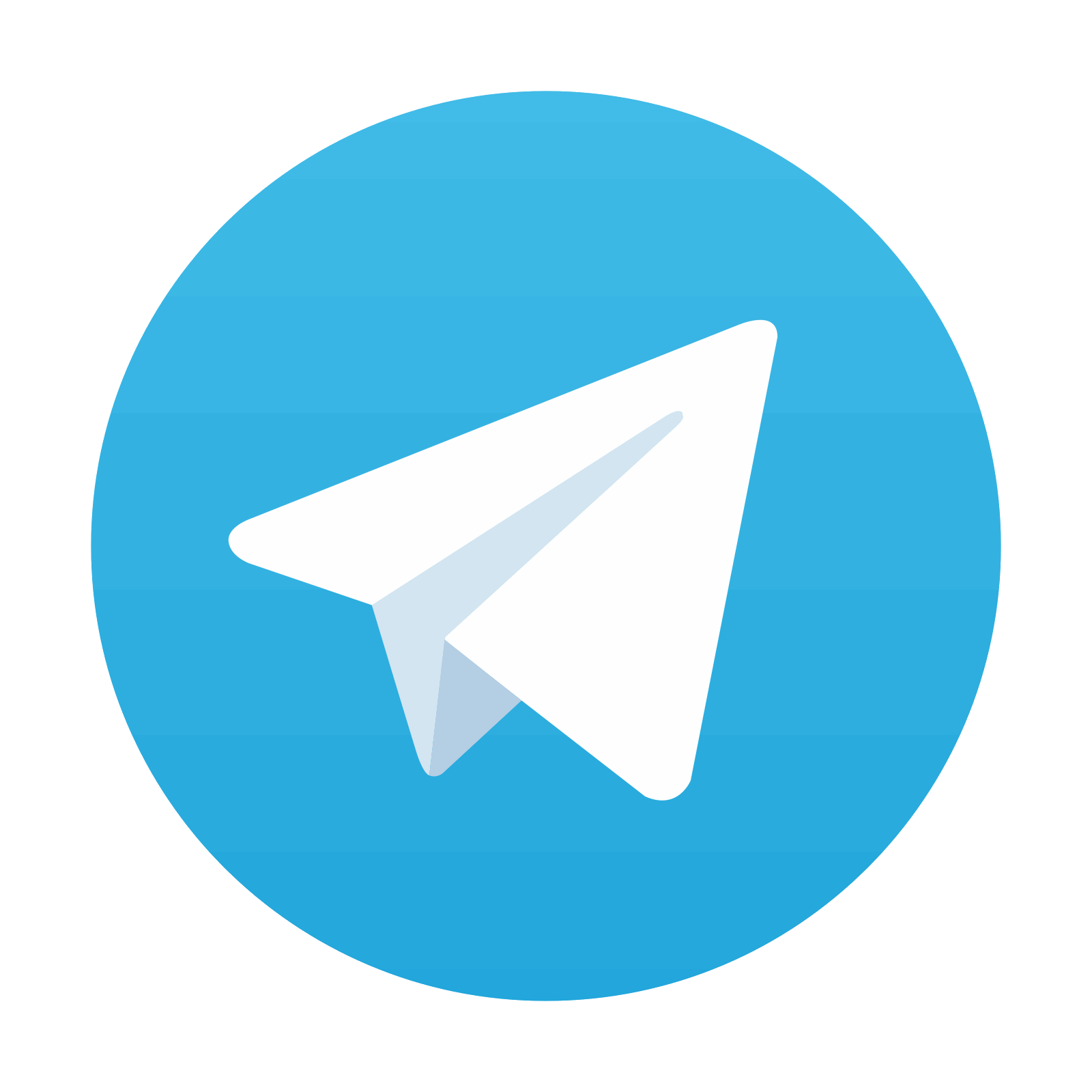
Stay updated, free articles. Join our Telegram channel

Full access? Get Clinical Tree
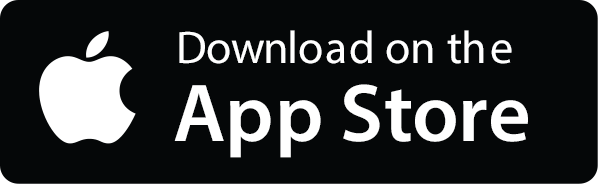
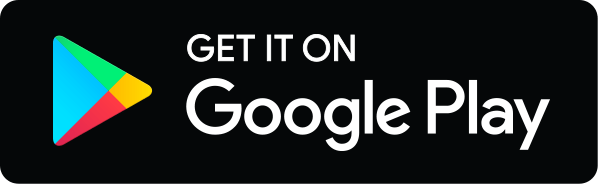