2
Amino Acid Neurotransmitters: Glutamate, GABA, and the Pharmacology of Benzodiazepines
Thomas F. Murray
Creighton University, Omaha, NE, USA
Introduction
In addition to their role in intermediary metabolism, certain amino acids function as small molecule neurotransmitters in the central and peripheral nervous systems. These specific amino acids are classified as excitatory or inhibitory, based on the characteristic responses evoked in neural preparations. Application of excitatory amino acids such as glutamic acid and aspartic acid typically depolarize mammalian neurons, while inhibitory amino acids such as gamma aminobutyric acid (GABA) and glycine characteristically hyperpolarize neurons. Glutamate, aspartate, and GABA all represent amino acids that occur in high concentrations in the brain. The brain levels of these amino acid transmitters are high (μmol g−1) relative to biogenic amine transmitters (nanomol g−1) such as dopamine, serotonin, norepinephrine, and acetylcholine. In mammals, GABA is found in high concentrations in the brain and spinal cord, but is present in only trace amounts in peripheral nerve tissue, liver, spleen, or heart (Cooper et al. 2003). These observations reveal the enrichment of this amino acid in the brain and suggest an important functional role in the central nervous system (CNS).
Glutamatergic Synapses
Glutamate and aspartate produce powerful excitation in neural preparations and glutamate is generally accepted as the most prominent neurotransmitter and major excitatory transmitter in the brain. The establishment of glutamate as a neurotransmitter in the brain was historically difficult due to its role in general intermediary metabolism. Glutamate is also involved in the synthesis of proteins and peptides, and also serves as the immediate precursor for GABA in GABAergic neurons. The enzyme glutamic acid decarboxylase (GAD) converts glutamate to GABA in these GABAergic neurons. In contrast to GABA, the glutamate content of brain outside of glutamatergic neurons is high as a consequence of its role in intermediary metabolism and protein synthesis. An array of neurochemical methods accordingly has demonstrated that all cells contain some glutamate, and in the brain all neurons contain measurable amounts of glutamate.
In neurons, glutamate is primarily synthesized from glucose through the pyruvate→acetyl‐CoA → 2‐oxoglutarate pathway, and from glutamine that is synthesized in glial cells, transported into nerve terminals, and converted by neuronal glutaminase into glutamate. In terminals of glutamatergic neurons, glutamate is stored in synaptic vesicles from which Ca2+‐dependent release in response to depolarization occurs. This synaptically released glutamate is taken up, in part, by glial cells and converted to glutamine by the enzyme glutamine synthetase. This glutamine is then transported back to neurons where glutamate is regenerated through the action of glutaminase (Figure 2.1).

Figure 2.1 Schematic representation of a glutamatergic synapse. Glutamine (Gln) is converted to glutamate (Glu) by mitochondrial glutaminase in glutamatergic neurons. Glutamate is released into the synaptic cleft where it may activate both pre‐ and postsynaptic receptors. Glutamate in the synaptic cleft is recaptured by neuronal and glial plasma membrane transporters that terminate the synaptic actions of the excitatory transmitter. Glial glutamate is converted to glutamine by the enzyme glutamine synthetase. This glutamine is then shuttled back to glutamatergic neurons to replenish the glutamate. Glutamate receptors include both G‐protein coupled (mGluRs) and ligand‐gated ion channel (AMPA, NMDA and kainite) receptors.
Extracellular glutamate concentrations are maintained within physiological levels by a family of transmembrane proteins known as excitatory amino acid transporters (EAATs). At least five EAATs have been identified with individual subtypes, differing with respect to their pharmacology and distribution within the CNS. Two of these EAATs are localized primarily on glial cells in the CNS with the other three EAAT subtypes being localized to neurons. The two glial glutamate transporters have been shown to be the primary regulators of extracellular glutamate in the CNS (Amara and Fontana 2002). EAATs accomplish glutamate influx driven by the cotransport of 3 Na+ and 1 H+ ions with the countertransport of 1 K+ ion. EAAT‐mediated glutamate uptake is therefore electrogenic and dependent on the Na+ gradient. The 3:1 ratio of Na+ to glutamate molecules transported causes a significant Na+ influx into the glial cells when the glutamate uptake is stimulated (Kirischuk et al. 2016). This elevation of intracellular Na+ can trigger a reverse mode of action of the Na+/Ca2+ exchanger (NCX), leading to a stimulation of Ca2+ signaling pathways in glia or neurons.
There is some evidence that neuronal EAATs are localized predominantly outside the synapse where they control extrasynaptic, rather than synaptic, glutamate concentration. This extrasynaptic glutamate may function to activate pre‐ and postsynaptic metabotropic glutamate receptors (mGluRs). Presynaptic mGluRs are involved in the feedback regulation of synaptic glutamate release.
In the normal brain, the prominent glutamatergic pathways are: (i) the cortico‐cortical pathways; (ii) the pathways between the thalamus and the cortex; and (iii) the extrapyramidal pathway (the projections between the cortex and striatum). Other glutamate projections exist between the cortex, the substantia nigra, the subthalmic nucleus, and the pallidum. Glutamate‐containing neuronal terminals are ubiquitous in the CNS and their importance in brain function and neurotransmission is therefore considerable. Estimates of the fraction of neurons in the brain that use glutamate as a neurotransmitter range from 70% to 85%.
Glutamate receptors are categorized into two main classes, namely, ionotropic glutamate receptors (iGluRs) and metabotropic receptors (mGluRs). The iGluRs were originally classified using a pharmacologic approach that led to identification of three subtypes bearing the names of selective agonists: (i) the AMPA; (ii) kainate; and (iii) NMDA receptors. These glutamate receptor subtypes are often described as being either NMDA (N‐methyl‐D‐asparate) or non‐NMDA (AMPA and kainate) receptors based on their sensitivity to the synthetic aspartate analog NMDA. All of these iGluRs represent ligand‐gated cation channels permeable to Na+ and K+ with differing permeabilities to Ca2+. Activation of these receptors by glutamate or selective agonists at normal membrane potentials allows Na+ to enter the cell with attendant membrane depolarization; this is the underlying mechanism for the rapid excitatory response of most neurons to glutamate. In addition to Na+ permeability NMDA receptors also have a high permeability to Ca2+ and display a voltage‐dependence of inward currents carried by Na+ and Ca2+ ions. The voltage dependence of the inward ionic current through the NMDA receptor arises from Mg2+ blockade of this channel at normal resting membrane potentials. This channel‐blocking action of extracellular Mg2+ is relieved when the cell is depolarized. Thus, the NMDA receptor signaling requires depolarization of the cell through the excitatory actions of non‐NMDA receptors before this ligand‐gated ion channel can produce an inward current. This property of NMDA receptors has led to the channel being termed a coincident detector due to the requirement for simultaneous activation of NMDA receptors and excitatory input to a cell as a precondition for the passage of ionic current through NMDA receptor ion channels.
A molecular classification of glutamate receptors has confirmed the subdivision based on pharmacological profiles of receptor subtypes. Molecular cloning techniques have identified gene families corresponding to each functional subtype of glutamate receptor. NMDA receptors are formed by assemblies of three gene families including NR1, NR2A‐D, and NR3A/3B (Mayer and Armstrong 2004). Functional NMDA receptors exist as heteromers containing two NR1 and two NR2 subunits (Erreger et al. 2004). NMDA receptors can also contain NR3A subunits that modulate the channel function. AMPA receptors are comprised of assemblies from the GluR1–GluR4 gene family, whereas kainate receptors are assemblies of GluR5–GluR7 and KA1 and KA2 subunits.
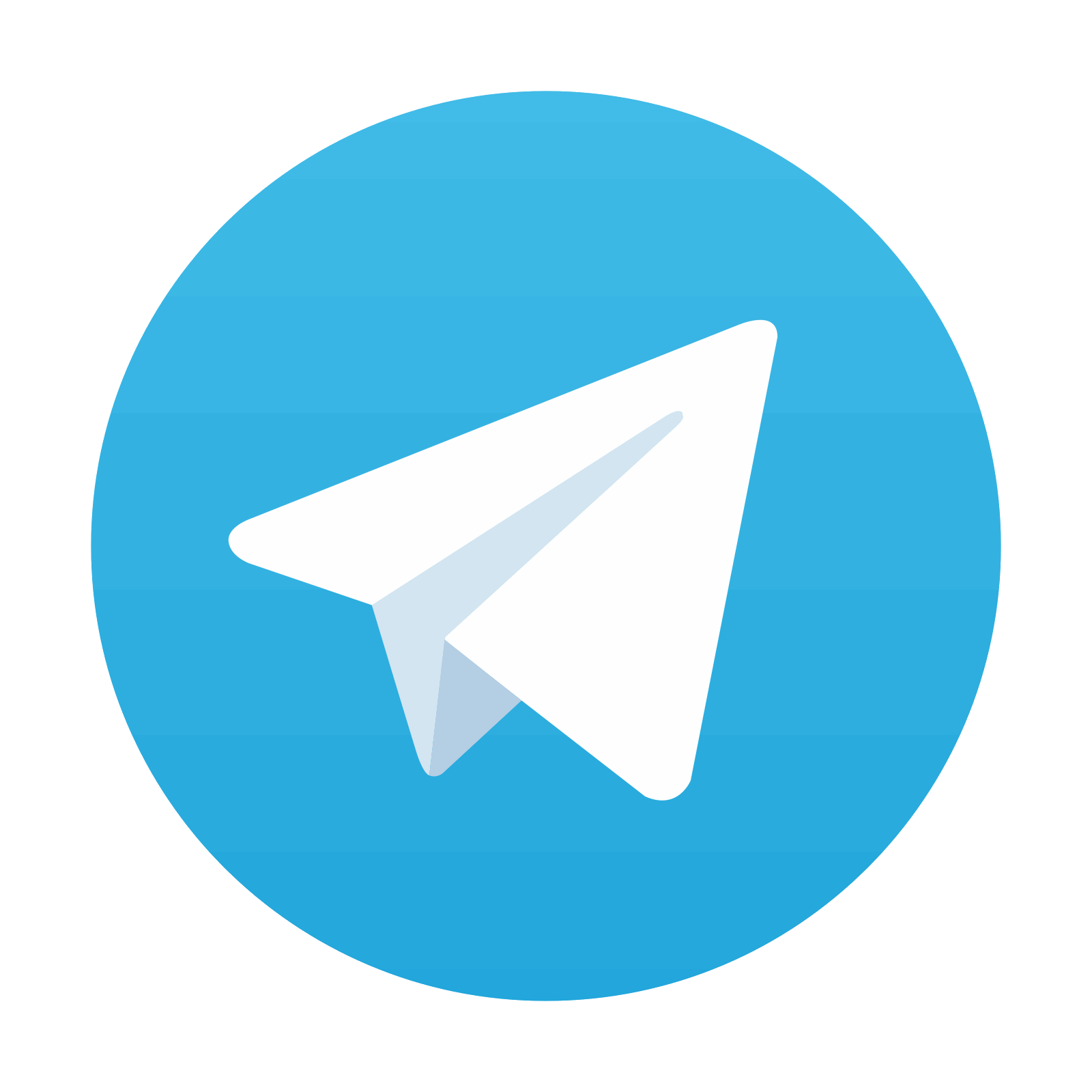
Stay updated, free articles. Join our Telegram channel

Full access? Get Clinical Tree
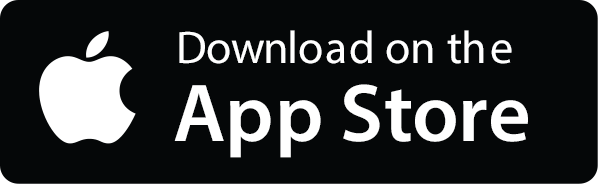
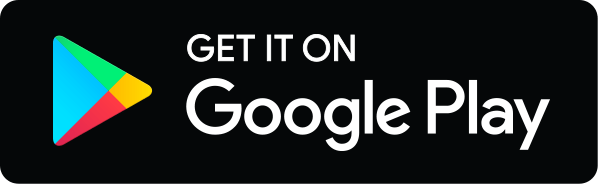