Chapter 7
Alternative Plasma Protein Products: Albumin and Human Immunoglobulin Therapy
Nicole Spurlock
Small Animal Specialist Hospital, North Ryde, New South Wales, Australia
Introduction
The field of veterinary transfusion medicine is rapidly expanding beyond replacement of basic blood components and is moving to target supplementation of more specific molecules. As we develop a broader understanding of physiology, immunology, and critical care, the isolation and transfusion of specific plasma proteins becomes increasingly desirable. Albumin and immunoglobulin replacement therapy is considered commonplace in human medicine and has been for decades. Historically, transfusion of these proteins has been less available to the veterinary community as only human products have been available. Although xenoprotein transfusion carries increased risks over standard transfusion procedures, there is a growing body of literature to support the use of human protein transfusion to companion animals. Furthermore, some animal-specific proteins are available and decrease the risk associated with therapy.
This chapter provides a summary of the body of information describing the use of alternative plasma proteins in veterinary medicine, focusing on albumin transfusion and human intravenous immunoglobulin (hIVIG) therapy in companion animals. The physiologic role of plasma proteins, adverse effects of therapy, and applications for veterinary patients are reviewed.
Albumin
The albumin molecule plays an integral role in both health and disease states (Box 7.1). This protein is most widely known for its importance in maintaining colloid osmotic (oncotic) pressure (COP), but albumin also functions as a carrier of drugs and exogenous substances, an antioxidant, a modulator of coagulation, and a buffer in the extracellular fluid. Albumin also has immunoprotective properties and helps to maintain endothelial cell viability (Mathews 2008).
Structure and function: colloid osmotic pressure
Albumin is a small but highly charged molecule with a molecular weight of 69 kD. Although it represents 50–60% of all plasma proteins in the body, it is responsible for generating 80% of COP in healthy animals (Mathews 2008). COP is one of the forces preventing fluid from exiting the intravascular space and moving into the interstitium. COP is generated by macromolecules located in the intravascular space, most importantly albumin. Albumin’s large contribution to COP is mostly due to Van’t Hoff’s law, which states that the COP generated by a particle is indirectly proportional to its molecular weight. In other words, the smaller the particles contained within the solution, the higher the COP of the solution. The strong charge of the albumin molecule also plays a smaller role in the generation of COP as described by the Gibbs–Donnan effect: the negative charge of the protein attracts sodium cations, which in turn attract water across the semipermeable vascular endothelial membrane (Figure 7.1). Together, the size and charge of the albumin molecule create the majority of the COP in the intravascular space and help to ensure that fluid stays within the vasculature (Throop et al. 2004).

Figure 7.1 The Gibbs–Donnan effect describes how molecular charge can generate an increase in intravascular colloid osmotic pressure. Intravascular albumin molecules do not cross the endothelial membrane during health. The negatively charged albumin proteins attract sodium cations, which move across the semi-permeable endothelium into the vascular space. Water molecules follow sodium cations across the endothelial membrane and lead to an increase in intravascular volume. (Illustration courtesy of Brandon Mugar.)
Carrier function and antioxidant properties
Albumin molecules have a strong binding capacity for many endogenous and exogenous substances (Table 7.1). Albumin functions as a carrier protein for many pharmacologic agents and effectively transports several drugs to sites of action, metabolism, or excretion. That same carrying capacity allows albumin to further function as a drug reservoir because substances that are protein bound are not free to interact with target tissues. Conversely, hypoalbuminemic patients will have an increased level of unbound agents circulating that are free to generate physiologic action. Increased availability of active drug compounds can result in toxicity and also decrease the drug half-life. As such, dose reduction should be considered when administering albumin-bound drugs to hypoalbuminemic patients (Ikenoue et al. 2000). Unfortunately, a predictable relationship between albumin concentration and pharmacologic action does not exist, and choosing appropriate dose alteration can prove difficult. Currently, no guidelines exist regarding dose reduction of protein-bound medications in hypoproteinemic patients (Koch-Weser and Sellers 1976; Ikenoue et al. 2000).
Table 7.1 Substances bound by albumin (Mazzaferro et al. 2002; Mathews 2008)
Endogenous | Exogenous |
Arachidonic acid | Amitriptyline |
Bilirubin | Cephalosporins |
Calcium | Diazepam |
Cysteine | Digoxin |
Fat-soluble vitamins | Furosemide |
Fatty acids | Glipizide |
Glucocorticoids | Hydralazine |
Iron | Ibuprofen |
Reactive oxygen species | Penicillins |
Thyroxine | Phenobarbital |
Tryptophan | Propanolol Radiocontrast iodine Salicylic acid Sulfonamides Tetracyclines Thiopental Warfarin |
Endogenous material is also bound by albumin. Many harmful bacterial toxins and carcinogens are neutralized when binding to the protein, although the albumin molecule is often destroyed as a result. Albumin also exhibits antioxidant properties and is thought to limit ischemia-reperfusion damage to inflamed tissue. By directly scavenging reactive oxygen species and binding iron molecules, albumin prevents iron-dependent lipid peroxidation and truncates oxidative damage (Watts and Maiorano 1999).
Coagulation mediation
The albumin molecule plays a role in coagulation by binding arachidonic acid and enhancing antithrombin (AT) activity (Doweiko and Bistrian 1994). In basic terms, arachidonic acid molecules are metabolized into prostaglandins, which activate platelets that aggregate and cause thrombosis. Low serum albumin concentrations result in higher levels of circulating arachidonic acid and a prothrombotic state. Furthermore, albumin demonstrates heparin-like activity by enhancing AT activity in vitro (Jørgensen and Stoffersen1979; Doweiko and Bistrian 1994). The combination of increased platelet aggregation and decreased anticoagulant activity in hypoalbuminemic patients might contribute substantially to thromboembolic events in the critically ill (Jørgensen and Stoffersen1979).
Miscellaneous functions
Beyond those already described, the albumin molecule fulfills a multitude of other roles in the body (Figure 7.2). Albumin acts as a buffer in the extracellular fluid compartment and provides amino acids for protein synthesis in catabolic states (Throop et al. 2004). It exhibits immunoprotective activity by inhibiting endothelial cell apoptosis and limiting activation of inflammatory cytokines (Kremer et al. 2011). Additionally, albumin supports microvascular integrity by occupying space in vessel walls and repelling macromolecules (Emerson 1989). Finally, albumin plays a role in wound healing. When the albumin protein extravasates from “leaky” vessels to sites of inflammation, it carries with it substrates vital for collagen crosslinking and tissue repair (Mazzaferro et al. 2002).

Figure 7.2 The albumin molecule has many roles in human and veterinary physiology. Egg whites are made primarily of the albumin protein.
Synthesis and distribution
Albumin is synthesized in the liver in response to low COP and hypoalbuminemia. Synthesis is influenced by several factors, including nutritional status, serum electrolyte concentrations, and hormonal balance (Beathard 1975). Simply stated, albumin production begins after a significant decrease in COP is detected at the level of the hepatic interstitium. This generally occurs when albumin levels are at 33% of maximum capacity and when adequate nutrients are available. Albumin has a serum half-life of approximately 8 days in healthy dogs, but is slightly prolonged in states of hypoalbuminemia (Powell 2008).
With regards to total body albumin, 40% is located in the intravascular space and 60% is present in the interstitial compartment (Mathews 2008). During health, there is constant flux between the two compartments, at a rate of 4% hourly. During times of acute protein loss or decreased synthesis, a more rapid exchange occurs between the two compartments until the extravascular supply is depleted (Doweiko and Nompleggi 1991). The increased exchange rate helps to preserve COP in the short term, but is not sustainable as there is no true storage pool for albumin in the body. Importantly, measured serum albumin concentrations might not accurately reflect total body depletion because of the flux between the intra- and extracellular spaces (Mathews 2008).
Causes and consequences of hypoalbuminemia
Hypoalbuminemia is almost always a component of critical disease, secondary to increased loss and degradation, decreased synthesis, and increased use during catabolic states (Box 7.2). Albumin can be lost via the kidneys or gastrointestinal tract. Extravasation can also occur secondary to vascular leak from damaged or inflamed endothelium. Circulating toxins contribute to destruction of available albumin and protein can also be denatured by cytokines present in inflamed tissue.
Decreased synthesis of albumin can also significantly contribute to hypoalbuminemia. Albumin is a negative acute phase protein: during times of metabolic stress or illness, albumin synthesis is downgraded as cytokines shunt amino acids towards production of acute phase proteins vital to the inflammatory process (Mathews 2008). Liver insufficiency can also contribute to low serum albumin. Although albumin concentrations are sometimes used as a marker of liver function, plasma albumin concentration will remain normal until liver function decreases below 10–25% (Beathard 1975). During end-stage liver disease, low protein concentration occurs not only as a result of decreased production, but also as a sequela of third spacing into ascites.
Although a mild decrease in serum albumin concentration results in few clinical concerns, moderate hypoalbuminemia compromises hemostasis and can result in edema, decreased perfusion, and tissue hypoxia. If vascular integrity is intact, fluid extravasation rarely occurs when serum albumin concentrations are over 1.5 g/dL (15 g/L) (Willard and Tvedten 2012). However, systemic inflammation from any cause can lead to endothelial compromise and vascular leak, thus exacerbating the effects of existing albumin deficits. Hypoalbuminemic patients are at high risk of hypotension, hypoperfusion, and multiple organ dysfunction syndrome. Edema and fluid accumulation within body cavities occurs, leading to a variety of complications; these are largely dependent on the location and extent of fluid accumulation and can include discomfort, decreased gastrointestinal absorption, gastrointestinal ileus, feeding intolerance, ascites, intra-abdominal hypertension, pulmonary edema, pleural effusion, respiratory compromise, delayed wound healing, and surgical dehiscence (Doweiko and Nompleggi 1991; Woods and Kelley 1993). As previously discussed, moderately to severely hypoalbuminemic patients are also prone to thromboembolic events (Jørgensen and Stoffersen 1979; Doweiko and Nompleggi 1991).
Albumin transfusion in human medicine
Despite the intuitive benefits of albumin transfusion for the support of protein-depleted patients, the efficacy of albumin supplementation in human medicine has faced significant debate. Multiple studies have been published with differing outcomes and a consensus regarding albumin supplementation has not been reached. The benefits of albumin transfusion appear to vary between population subsets and more research is needed before firm conclusions can be drawn regarding the practice of albumin transfusion in the critically ill.
Fluid resuscitation
Although not often used for fluid resuscitation in veterinary patients, 4% albumin has historically been employed as a resuscitative fluid in human patients. A commonly cited meta-analysis conducted in 1998 examined a large number of heterogeneous studies and concluded that critically ill human patients receiving 4% or 5% albumin solution for volume replacement had a 6% increase in mortality compared to those patients not receiving albumin (Cochrane Inquiries Group Albumin Reviewers 1998). Many clinicians questioned the conclusions of the group and further studies were subsequently conducted evaluating the use of albumin in human patients.
The Saline versus Albumin Fluid Evaluation (SAFE) study was published in 2004 and was designed to evaluate risk in human patients administered 4% albumin versus 0.9% NaCl during fluid resuscitation. Initial albumin concentrations or reasons for hospital admission were not recorded. In direct contrast to the original meta-analysis, administration of albumin for volume replacement in critically ill patients did not result in increased mortality. In fact, both protocols resulted in equivalent death rates within the 28-day trial period (Finfer et al. 2004).
In 2012, another meta-analysis compared multiple randomized clinical trials and evaluated outcome in critically ill patients receiving different colloid solutions for fluid resuscitation. Although the analysis found no increase in mortality associated with albumin administration, no clear benefits could be demonstrated with albumin supplementation in the overall patient population. However, the evidence suggested that patient response to albumin therapy varied between specific patient populations. The authors concluded that while albumin therapy might be beneficial during resuscitation of septic patients, it might increase mortality in patients suffering from burns, traumatic brain injury, or hypoalbuminema (Bunn and Trivedi 2012). To date, a consensus has not been reached on the use of albumin-containing fluids during fluid resuscitation of critically ill patients, as subsequent studies have not found significant advantages with the use of albumin over crystalloids (Perel et al. 2013).
Septic patients
The trend towards improved survival after albumin administration in septic patients raises the possibility that albumin might be beneficial in this population. A meta-analysis comparing the use of albumin versus crystalloids for fluid resuscitation of human patients with severe sepsis or septic shock demonstrated a reduction in 90-day mortality in patients with septic shock that were administered albumin (Xu et al. 2014). However, another meta-analysis was unable to demonstrate any advantage of using albumin-containing fluids for resuscitation of patients with sepsis of any severity (Jiang et al. 2014).
Unfortunately, because albumin is so much more expensive than crystalloids, it can be difficult to justify its use as a first-line resuscitative fluid. However, albumin supplementation might improve outcome in septic patients when used as an adjunct to standard resuscitative therapy. Current Surviving Sepsis Campaign Guidelines recommend crystalloids as the initial fluid choice in the resuscitation of sepsis and septic shock, but advise addition of albumin to the treatment protocol for patients who persistently require high-volume crystalloid therapy to maintain adequate mean arterial pressure (Dellinger et al. 2013).
Acute lung injury and acute respiratory distress syndrome
Hypoproteinemia is one of the strongest independent predictors for the development of acute lung injury (ALI) and acute respiratory distress syndrome (ARDS) in the critically ill, and several studies have focused on the benefit of albumin therapy in this subset of patients. One such study investigated the use of furosemide and albumin in patients with ALI or ARDS. Decreased morbidity and fewer days of mechanical ventilation occurred in hypoalbuminemic patients who received a 5-day standardized regimen of 25% albumin and furosemide. The authors concluded that albumin and furosemide therapy decreases pulmonary edema and improves fluid balance, oxygenation, and hemodynamics in patients with ALI (Martin et al. 2002). A follow-up study was conducted comparing the administration of furosemide and albumin to furosemide therapy alone. Combination therapy was more effective as patients receiving albumin had greater increases in oxygenation and serum protein concentrations (Martin et al. 2005). Improvement in overall mortality has not been demonstrated at this stage, but emerging trends suggest that albumin therapy might be beneficial in patients with ALI or ARDS (Martin et al. 2005; Calfee and Matthay 2007).
Current human recommendations
Although promising new research is being generated, the controversy regarding albumin transfusion in human medicine continues. Albumin is not currently recommended as a standard resuscitation fluid for all patients as no clear benefit has been demonstrated when used in this capacity. Additionally, existing studies do not clearly demonstrate a decrease in morbidity and mortality associated with albumin supplementation in non-resuscitative situations (i.e., as maintenance fluids), and the cost of therapy is significantly higher when compared to synthetic colloids. Serious adverse effects are rare in humans receiving albumin and occur with an incidence of 1.29 per 106 doses (Vincent 2003). Regardless, data garnered from current literature is inadequate to justify routine administration of albumin to all hypoalbuminemic critically ill patients. However, the use of albumin transfusion might be advantageous in specific patient populations and is generally considered a reasonable adjunct to standard therapy.
Indications for albumin therapy in veterinary patients
Administration of albumin-containing solutions is not warranted for all critically ill patients and should be used conservatively in hypoalbuminemic patients. A niche population of animals might benefit from albumin therapy, but each patient must be considered individually prior to transfusion. Current research suggests that albumin supplementation could be considered in hypoalbuminemic dogs with serum albumin concentrations <2.0 g/dL (20 g/L). Septic patients or those with vasculitis, systemic inflammatory response syndrome (SIRS), or edematous manifestations of disease might also benefit from albumin therapy (Mathews and Barry 2005). Despite the potential benefits associated with therapy, note that critical patients with ongoing refractory protein loss (e.g., protein losing enteropathy or nephropathy [PLE/PLN]) might quickly lose any albumin transfused (Mathews 2008). Likewise, albumin products are not appropriate for routine fluid resuscitation and should not be used to increase serum albumin concentrations >2.0–2.5 g/dL (20–25 g/L) so as not to suppress endogenous albumin production (Barton 2005; Mathews 2008). Albumin transfusion can be considered as an adjunct to standard therapeutics and might improve global perfusion and oxygen delivery in hypoproteinemic patients.
Albumin supplementation in small animals
In veterinary patients, intravenous albumin can be provided in three forms: species-specific plasma, human serum albumin (HSA), or canine-specific albumin (CSA). While synthetic colloid administration is effective for support of COP, hydroxyethyl starches do not possess the same physiologic benefits of albumin and have associated risks such as coagulopathies and acute kidney injury (Myburgh et al. 2012). The use of species-specific plasma is generally not recommended as a method for resolution of hypoalbuminemia due to the large volume of product required, associated cost of administration, and added risk of transfusion-associated complications. In order to increase the plasma albumin concentration by 0.5 g/dL (5 g/L) in a hypoalbuminemic dog, a minimum dose of 22.5 mL/kg of plasma is needed (Throop et al. 2004).
Human serum albumin in canine patients
The body of literature describing the use of HSA for transfusion in veterinary patients is limited, but several studies documenting the use of HSA transfusion in healthy and critically ill veterinary patients are available (Table 7.2). Early studies focused on transfusion of 25% HSA to combat conditions including sepsis, peritonitis, trauma, neoplasia, pancreatitis, gastric dilation volvulus, hepatic disease, PLE/PLN, gastric ulceration, hypotension, and hypoalbuminemia (Figure 7.3). In these studies, serum albumin concentrations and total protein measurements were up to 45% higher post-transfusion (or at discharge) in most patients. Only subjects in the PLE/PLN group showed decline in albumin levels post-transfusion, indicating that HSA transfusion offers only transient benefit in animals suffering from ongoing protein loss. Almost no adverse effects were noted in transfused animals and the authors concluded that HSA can be safely administered to select critically ill animals and might improve outcome (Chan et al. 2004; Mathews and Barry 2005; Mathews 2008).
Table 7.2 Albumin use in veterinary patients
Condition | Concentration | Dose |
Hypotension | 25% HSA | 500 mg/kg to 1 g/kg slow bolus followed by 25–425 mg/kg/hour over 4–72 hours (Mathews 2008) |
Hypoalbuminemia | 25% HSA | 0.1–1.7 mL/kg/hour over 4–72 hours Maximum volume administered 25 mL/kg (Mathews 2008) |
Critically ill population of dogs: sepsis, peritonitis, trauma, neoplasia, pancreatitis, gastric ulceration | 25% HSA | Total dose of 380 mg/kg to 3.6 g/kg (Chan et al. 2004) |
Critically ill population of dogs: septic peritonitis, trauma, neoplasia, hepatic disease, gastric ulceration | 25% HSA diluted to 10% | Total dose of 100 mg/kg to 6 g/kg (Trow et al. 2008) |
Critically ill population of dogs and cats: GDV, peritonitis, nephropathy, pancreatitis, hepatic disease, PLE* | 25% HSA diluted to 5% | 100 mg/kg/hour for 10 hours, administered daily until serum albumin reached 2.0 g/dL (20 g/L) (Vigano et al. 2010) |
PLE and PLN | 25% HSA | Total dose of 200 mg/kg to 4.2 g/kg (Mathews and Barry 2005) |
Liver disease* | 25% HSA | Total dose of 350 mg/kg to 6.3 g/kg (Mathews and Barry 2005) |
GDV | 25% HSA | Total dose of 475 mg/kg to 1.7 g/kg (Mathews and Barry 2005) |
Septic peritonitis, hypoalbuminemia | 5% CSA | Total dose of 800–884 mg/kg over 6 hours (Craft and Powell 2012) |
GDV, gastric dilation volvulus; PLE, protein losing enteropathy; PLN, protein losing nephropathy; HSA, human serum albumin; CSA, canine-specific albumin.
* Indicates studies that include cats, other studies include dogs only.

Figure 7.3 Albumin transfusions can be advantageous to treat a myriad of diseases. This patient received an albumin transfusion, in addition to standard therapies, for the management of septic peritonitis.
More recent studies have evaluated the application of 5–10% HSA transfusion in critically ill patients with the same conditions outlined above. Potential benefits of transfusion with more dilute HSA include provision of a more physiologic approach to albumin supplementation, decreased risk of volume overload, and development of fewer transfusion reactions. As with administration of 25% HSA solutions, significant increases in serum albumin levels were achieved with transfusion of 5% and 10% solutions. No adverse effects were reported when an isotonic (5% HSA) solution was transfused. When administered 10% HSA, 6.8% of patients suffered severe complication peri-infusion (Trow et al. 2008; Vigano et al. 2010). Importantly, achievement of a serum albumin level of 2.0 g/dL (20 g/L) with 5% and 10% HSA administration appears to take up to 7–10 days compared to 1–2 days when 25% HSA solutions are administered.
Although these studies support the use of HSA transfusion as a safe and useful intervention in critically ill hypoalbuminemic animals, most research describing HSA use in veterinary patients is retrospective in nature and lacks patient follow-up. Caution should be used when interpreting available data and drawing practical conclusions.
Adverse effects in dogs
Significant concerns have been raised regarding adverse effects associated with the administration of a human protein to companion animals. As with any xenoprotein transfusion, there is an inherent risk of hypersensitivity after initial or repeated exposures. Several studies have demonstrated that the administration of HSA to healthy dogs results in non-immune anaphylaxis and type III hypersensitivity reactions (Cohn et al. 2007; Francis et al. 2007; Martin et al. 2008). Clinical signs of acute reactions during HSA transfusion include facial edema, tachypnea, vomiting, and fever. In some cases, manifestations of immune-mediated hypersensitivity appear 10 days to 2 months after transfusion and include polyarthritis, dermatitis, and vasculitis. Delayed (type III) hypersensitivity reactions after HSA transfusion in two healthy dogs even resulted in death (Francis et al. 2007). As a result of these studies, it is not recommended to administer HSA to healthy animals (Cohn et al. 2007).
Despite several documented and, even lethal, hypersensitivity reactions in healthy dogs administered HSA, studies document HSA transfusions in systemically ill veterinary patients occurring without incident (Chan et al. 2004; Matthews and Barry 2005).The dichotomy likely stems from the fact that immunosuppression is an integral component of critical disease. Every patient considered for albumin therapy in a clinical setting is ill and immunocompromised to some extent. It has been hypothesized that critically ill patients suffer fewer adverse effects after albumin transfusion because they are unable to mount an appropriate response against infusion of a foreign protein (Francis et al. 2007; Martin et al. 2008). While it is indisputable that sick animals tolerate xenotransfusion better than their healthy counterparts, caution is still warranted when considering HSA transfusion. Development of lesions consistent with type III hypersensitivity in two critically ill dogs after treatment with 25% HSA has been documented. Reactions were self-limiting in both dogs that were not seriously compromised (Powell et al. 2013). Altogether, HSA transfusion might provide significant benefit to critically ill veterinary patients, but concerning risks exist and should be considered prior to administration. Development of anti-HSA antibodies has been documented after albumin transfusion in both healthy and sick dogs (Martin et al. 2008). Although some studies document multiple HSA transfusions without complication, repeat infusion of HSA to canine patients more than 7 days after initial transfusion is not advised (Trow et al. 2008).
Available products
Human serum albumin products are created from ultrafiltered pooled plasma and are composed of highly soluble globular proteins (Figure 7.4). Solutions are available as 5% and 25% concentrations (Grifols Therapeutics Inc., Clayton, NC; CSL Plasma, Boca Raton, FL). 5% HSA is isotonic and has a COP of 20 mmHg, while 25% HSA is hypertonic with a COP of 200 mmHg. Most HSA products are stable for 3 years if stored at room temperature (Figure 7.5). Temperatures below freezing and above 30°C (86°F) damage the product and render it ineffective. Once opened, albumin vials should be used or discarded after 24 hours (Mazzaferro et al. 2002).

Figure 7.4 Human serum albumin has historically been used for transfusion in veterinary patients, but species-specific albumin products are becoming more widely available (i.e., canine, bovine, and equine albumin).

Figure 7.5 A bottle of 25% human serum albumin (AlbuRx®, CSL Behring LLC, Kankakee, IL) can be stored at room temperature. (Image provided by Kenichiro Yagi, BS, RVT, VTS (ECC, SAIM).)
Careful consideration of fluid balance and patient comorbidity is imperative when choosing an appropriate concentration: administration of 25% HSA will increase intravascular volume to four to five times that of the volume infused (Mazzaferro et al. 2002). In normovolemic hypoproteinemic patients, an iso-oncotic 5% albumin infusion might be considered to decrease the risks associated with acute intravascular volume expansion. However, there is some evidence that treatment with 5% HSA does not confer the same anti-inflammatory benefits noted after transfusion of 25% HSA. An experimental cell model demonstrates significant differences in neutrophil behavior after incubation in lactated Ringer’s solution (LRS), 5% HSA, and 25% HSA: inflammatory cytokine activation and oxidative burst are greater for neutrophils incubated with LRS or 5% HSA compared to those incubated in 25% HSA (Rhee et al. 2000).
Dosing protocols for veterinary patients
The recommended goal for albumin supplementation in veterinary patients varies, but most clinicians target a serum albumin concentration of 2.0 g/dL (20 g/L) (Rudloff and Kirby 1998; Mazzaferro et al. 2002). Several dosing strategies exist and no standard guidelines have been established (Table 7.2). Some clinicians advocate calculating a patient’s albumin deficit and replacing it over 6–72 hours (Box 7.3). Unfortunately, the kinetics of HSA in small animals are not well described and the half-life of transfused products is not known at this time.
Alternatively, a recommended dose of 25% HSA has been extrapolated from human studies at 2 mL/kg over 2 hours, followed by 0.1–0.3 mL/kg/hour thereafter. The total dose administered should be 2.5–5 mL/kg of 25% HSA and a daily dose of 2 g/kg should not be exceeded (Powell 2008). A retrospective study documented the administration of 5% HSA to hospitalized dogs at a rate of 2 mL/kg/hour over 10 hours for several days. Daily transfusion was continued until a serum albumin of 2.0 g/dL (20 g/L) was obtained. This dosing regimen was designed to slowly increase albumin concentrations in a more physiologic manner and to decrease the risk of fluid overload. The median number of days required for serum albumin concentrations to reach 2.0 g/dL (20 g/L) was 4. Severe hypersensitivity reactions were not documented in any patient record and all dogs received their entire transfusion without incident (Vigano et al. 2010).
Human serum albumin administration in feline patients
There is limited information regarding the use of albumin transfusion in cats. An early retrospective study included two cats in a population of 66 critically ill animals receiving 25% HSA, with one cat dying within 18 hours of transfusion (Mathews and Barry 2005). More recently, another retrospective study documented HSA use in 170 hypoalbuminemic cats. A 5% HSA infusion was administered at a rate of 2 mL/kg/hour for 10 hours daily until serum albumin concentrations reached 2.0 g/dL (20 g/L). The cats received HSA transfusions for a median of 4 days and 123 cats survived to discharge. Adverse effects of HSA transfusion were reportedly mild, with diarrhea, tremors, or hyperthermia occurring in 36% of cats (Vigano et al
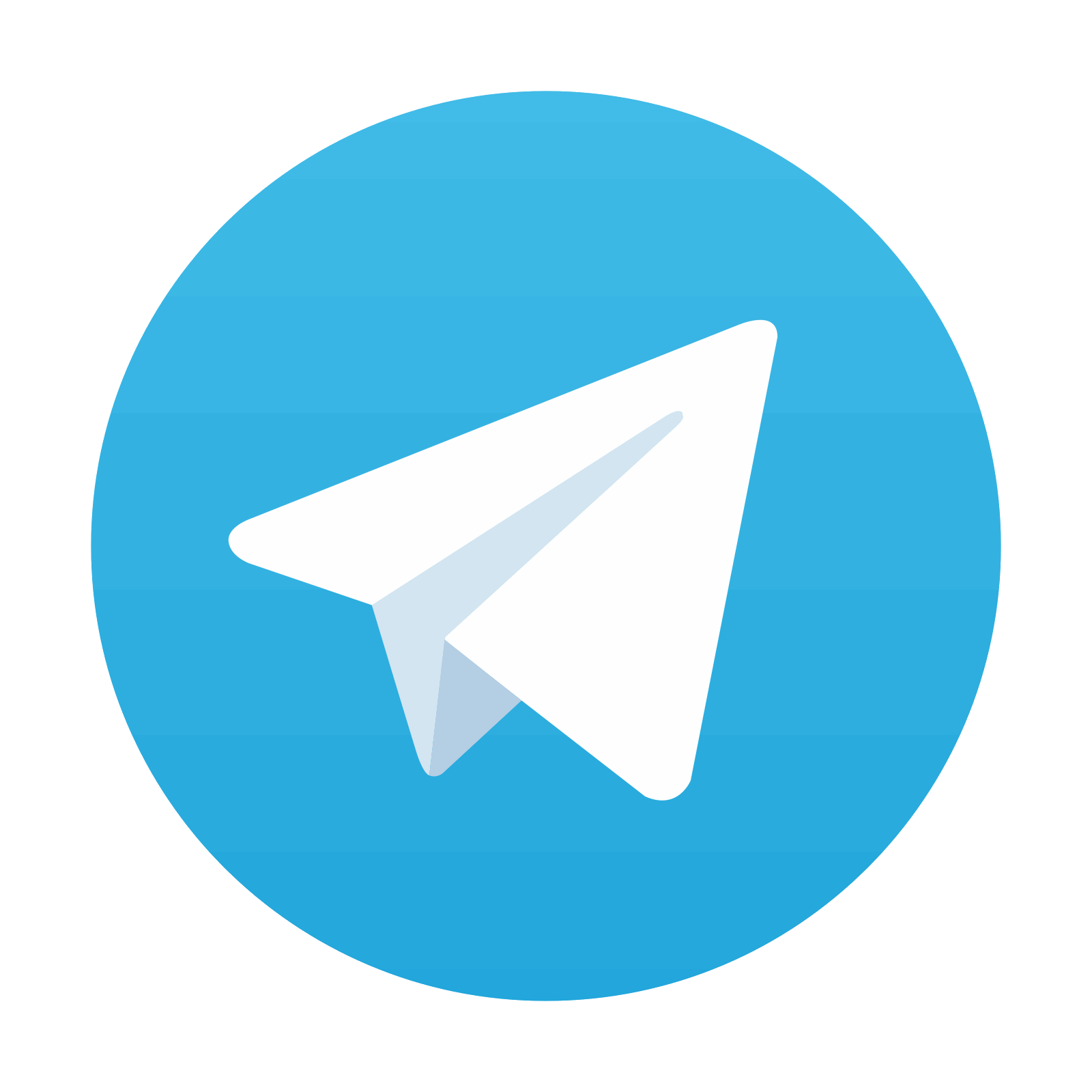
Stay updated, free articles. Join our Telegram channel

Full access? Get Clinical Tree
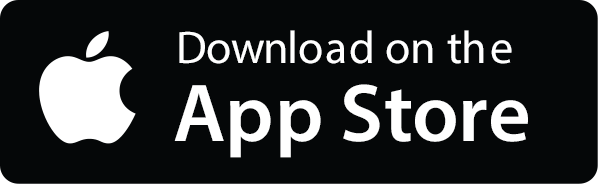
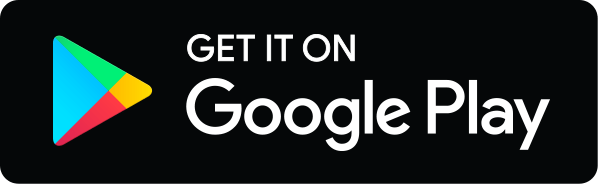