Andrea A. Bohn Department of Microbiology, Immunology, and Pathology, College of Veterinary Medicine and Biomedical Sciences Colorado State University, Fort Collins, CO, USA Evaluation of electrolyte concentrations and acid–base balance is typically used more to assess severity of disease and to guide treatment rather than as a diagnostic tool, although certain changes can aid in the diagnosis of some disorders. Abnormalities in electrolyte concentrations and acid–base balance are often associated with diarrhea, colic, rhabdomyolysis, prolonged exercise and sweating, renal disease, and endocrine abnormalities. This chapter will focus on acid–base and electrolyte changes commonly seen in horses and their causes. Maintaining the proper pH of blood and tissues is important for optimal enzymatic and cellular function. The normal pH range for horse blood is approximately 7.38–7.44. Below this range, the blood is acidemic and above the range it is alkalemic. Various processes are in place to help maintain normal pH. These involve the respiratory system, in which carbon dioxide (CO2) is exhaled, the kidneys, where excess acids or bases can be excreted, and buffer systems, the most important of which for our purposes of evaluation is bicarbonate (HCO3). An abnormality in acid–base balance is not a disease but a reflection of many possible disease processes. Evaluation of the acid–base status can give an indication that a serious problem exists. Acid–base abnormalities are traditionally described as being either a respiratory acidosis or alkalosis or a metabolic acidosis or alkalosis. A primary disorder within one of these systems typically results in a compensatory response in the other. Since the respiratory system is concerned with CO2 which is weakly acidic, and the metabolic system handles HCO3, which is a weak base, the movement of the compensatory response should be in the same direction (up or down) as the primary disorder. To determine which is the primary disorder, one must look at the blood pH. If the blood is acidemic, the system that is moving in the acidic direction is the source of the primary disorder. If the blood is alkalemic, the system that is moving in the alkaline direction has the primary disorder. If both branches are moving in an alkaline or acidic direction, there is a mixed acid–base disorder. Likewise, if the compensatory side has shifted more than expected for a compensatory response, there is likely a mixed acid–base disorder. In those cases, the pH may be normal, even with marked acid–base abnormalities. The following six‐point approach to acid–base status is very useful for a complete characterization. Bicarbonate and chloride (Cl) concentrations as well as AG are routinely provided within a serum biochemistry panel, which provides important information on the acid–base status of a horse. However, a complete characterization of acid–base status requires a full blood gas panel (preferably on arterial blood). AG, when increased, is always an indication of metabolic acidosis. Bicarbonate and Cl concentrations also reflect acid–base status, but whether abnormalities in these substances are due to primary metabolic derangements, compensatory mechanisms in response to respiratory acid–base imbalances, or there is a mixed acid–base disturbance often cannot be determined without also knowing the pH and pCO2 of the blood. Table 7.1 describes expected results for uncomplicated acid–base abnormalities. Bicarbonate is an important buffer in the blood and its concentration therefore reflects the acid–base status of an animal. Changes in HCO3 concentration can be due to metabolic derangements or compensation for respiratory acidosis or alkalosis. Bicarbonate concentration is typically reported either as total CO2 (TCO2), which is normally about 5% higher than HCO3, or as HCO3 concentration. In this chapter, bicarbonate will be designated as HCO3. Exposure of blood or serum to air can result in CO2 release, which causes underestimation of HCO3. In contrast, allowing blood to stand without exposure to air may result in overestimation of HCO3 due to metabolic processes. Therefore, appropriate handling of blood samples is an important step in laboratory analysis. With primary metabolic acid–base abnormalities, when AG is normal (no increase in organic acids) changes in HCO3 concentration are associated with a converse shift in Cl concentration. In this instance, the pH should reflect the HCO3 and Cl concentrations and if there is respiratory compensation, the pCO2 will have moved in the same direction (increased or decreased) as HCO3. To determine that there is a primary metabolic acidosis or alkalosis with respiratory compensation or vice versa, it is again necessary to evaluate the pH and pCO2. Hypochloremic metabolic alkalosis occurs when HCO3 is retained in the blood as Cl is lost from or sequestered within the upper gastrointestinal (GI) tract or lost from excessive sweating. Hyperchloremic metabolic acidosis occurs when Cl is retained in response to HCO3 loss from the intestinal tract or the kidneys (renal tubular acidosis). Table 7.1 Uncomplicated acid–base abnormalities and their expected changes. H, high; L, low; N, normal; AG, anion gap. Bicarbonate is a very important buffer for endogenous and exogenous acids. Endogenous acids include lactic acid and uremic acids; ketoacids are common in other species but horses are poorly ketogenic. Exogenous acids include metabolites of ethylene glycol and salicylate. An increase in any of these acids results in a decrease in HCO3 concentration as it buffers the hydrogen ion. While there are methods to measure many of the acids that cause a metabolic acidosis, an easy way to determine their presence is by calculating the AG. The AG is based on the fact that the numbers of negative and positive charges in plasma are equal. We do not routinely measure all substances in the blood and there are therefore many unmeasured anions and cations present. The commonly measured cations are sodium (Na) and potassium (K) and the commonly measured anions are Cl and HCO3. Because the sum of unmeasured and measured cations must equal the sum of unmeasured and measured anions in blood, we can determine the difference between the unmeasured components (AG) by calculating the difference between the measured components. And since the number of unmeasured cations usually does not change significantly, any abnormality in AG is related to the number of anions. A decrease in AG is associated with hypoalbuminemia since the predominant unmeasured anion in health is albumin. An increased AG indicates the presence of additional unmeasured anions in the blood; after HCO3 buffers the hydrogen ion from excess endogenous or exogenous acids, the corresponding anion is left in circulation. Chloride concentration does not change with high AG metabolic acidosis since the decrease in negative charge from HCO3 buffering is offset by the negative charge of the unmeasured anion present. Hypoalbuminemia can offset and mask the presence of other unmeasured anions when determined by AG. Respiratory acidosis typically occurs with respiratory depression or pulmonary disease and the resulting decrease in the amount of CO2 exhaled. Respiratory alkalosis occurs with hyperventilation (due to pain, anxiety, or iatrogenic means) as the result of increased amounts of CO2 being exhaled. The evaluation of the respiratory components of acid–base balance requires knowledge of blood pH and pCO2. Since pCO2 is acidic and HCO3 is basic, a primary increase in pCO2 is compensated by an increase in HCO3 and a primary decrease in pCO2 is compensated by a decrease in HCO3. When there is a primary respiratory acidosis (increased pCO2), HCO3 is retained by the kidneys to compensate for the acidemia. When there is a primary respiratory alkalosis (decreased pCO2), the kidneys increase HCO3 excretion in compensation. As HCO3 concentrations shift to compensate for respiratory acid–base imbalances, Cl concentrations shift in the opposite direction to maintain electrical neutrality. If there are abnormalities in the acid–base balance from metabolic processes, the respiratory system can act quickly to blow off either more or less CO2 to help maintain blood pH. This compensation can take place within minutes to hours. In general, for every 1 mEq of HCO3 abnormality, the respiratory system is able to adjust by about 0.7 mmHg CO2. The lungs blow off extra CO2 to compensate for metabolic acidosis and they retain CO2 to compensate for metabolic alkalosis. Several hours to days are needed for the kidneys to respond to respiratory acidosis or alkalosis. The kidneys eventually excrete an increased amount of HCO3 to compensate for respiratory alkalosis or retain an increased amount for respiratory acidosis. In respiratory acidosis, for every 10 mmHg increase in PCO2, the HCO3 concentration is expected to increase by 1–2 mEq in the acute phase and by 3–4 mEq if chronic. In respiratory alkalosis, for every 10 mmHg decrease in PCO2, the HCO3 concentration is expected to decrease by 2–3 mEq in the acute phase and by 4–6 mEq if chronic. Other buffers in the blood besides HCO3 include sulfates, phosphates, and hemoglobin, so the concentration of HCO3 does not always reflect the total buffering capacity of the blood. Base excess (BE) is a reported value that indicates how far off from the normal total buffering capacity an animal is. Another way to think of it is that BE indicates how much acid needs to be added in order to bring the pH back to normal. In health, the reference interval of BE is about −4 to +4. Numbers less than −4 indicate a deficiency in anionic buffers (a negative BE) and therefore an acidosis, while numbers greater than +4 indicate an excess of anionic buffers and the presence of an alkalosis that may require addition of acid to bring the pH back to normal. Sodium concentration and water balance are dependent on each other so when considering reasons for abnormalities in serum Na concentrations, one must also take into consideration water balance. Hydration status is typically determined by a combination of physical examination and laboratory assessments. Decreased skin turgor, increased capillary refill time, and tacky mucous membranes are subjective assessments of dehydration. Hemoconcentration is a result of dehydration that may be evidenced by detecting an increase in packed cell volume (PCV) and plasma protein concentration. Overhydration in horses is rare and typically does not occur unless there is decreased water excretion from the kidneys, which can be associated with renal, cardiac, or liver disorders. Psychogenic polydypsia can potentially lead to overhydration, but the renal system can usually handle increased water intake. Hypernatremia occurs if there is excess Na (rare) or decreased water content (Table 7.2). The potential for excess Na exists if animals have access to and ingest salt without having access to fresh water. Inappropriate administration of hypertonic fluids can also lead to Na excess. Most causes of hypernatremia include either pure water loss or loss of hypotonic fluids where water loss is greater than electrolyte loss. Table 7.2 Common causes of sodium and chloride abnormalities in horses. Pure water loss occurs with insensible water loss associated with evaporation from lungs and skin as well as fecal and urine water excretion. If insensible water losses are greater than water intake, serum Na concentration will increase. Increased water loss can occur with strenuous exercise, resulting in hypernatremia immediately post exercise. Decreased water intake can be due to an animal not having access to water or to a defective thirst response, as has been seen in horses with debilitating brain disease [1]. It must be remembered that serum Na concentration is related to the ratio of Na to water within vascular plasma and does not necessarily reflect the body’s Na content. Hypernatremia may be present when there is decreased water intake and/or increased water loss even though total body Na concentrations may be depleted from excessive loss during sweating [2–4]. With renal disease, there is often loss of electrolytes along with decreased urine concentrating ability, but when there is a defect primarily associated with concentration, as with either central or nephrogenic diabetes insipidus (lack of antidiuretic hormone [ADH] activity), hypotonic fluid loss and hypernatremia can occur. Hyponatremia most commonly occurs with loss of isotonic fluid and subsequent water repletion or, possibly, with loss of hypertonic fluid (more Na lost than water; see Table 7.2). Loss of isotonic fluid can result in hypovolemia, which stimulates renal water retention and thirst. Common sites of isotonic fluid loss include body cavities, intestinal tract, kidneys, and skin (sweating). Many different digestive system disorders cause isotonic fluid loss, including diarrhea, sequestration of fluid in the GI tract with ileus or obstruction, gastric reflux, or esophageal obstruction and loss of saliva. Renal failure or diuresis can lead to sodium wasting into the urine. Hyponatremia is also often seen with uroperitoneum since the urine leaking into the abdominal cavity is of low Na concentration, which rapidly equilibrates with plasma Na [5]. Third‐space fluid accumulation can lead to hyponatremia because of electrolyte and fluid shifts; hyponatremia can become pronounced with repeated removal of isotonic fluid from body cavities. If there is increased water intake and an ADH response, dilutional hyponatremia may ensue. Severe hypoalbuminemia, liver disease, or cardiac disease leading to third‐spacing of fluids (edema) may result in mild hyponatremia due to water retention in excess of Na retention, especially if total body Na stores are decreased. Equine sweat contains a Na concentration that is equal to or slightly higher than that of blood [6] so sweat can be a source of significant Na loss, particularly with exercise. At high ambient temperatures, horses at rest are typically able to maintain normal Na levels but after an endurance race, plasma sodium concentrations were shown to drop [7]. Less common reasons for hyponatremia include iatrogenic hypoadrenocorticism, excess administration of Na‐poor fluids, excessive water consumption, syndrome of inappropriate ADH secretion, and pseudohyponatremia. Pseudohyponatremia can occur if there are other substances causing hyperosmolality and drawing water into the vascular space, such as marked hyperglycemia or mannitol. Marked hyperlipidemia or hyperproteinemia can also result in pseudohyponatremia on some chemistry analyzers by taking up space within the plasma volume; using an ion‐specific electrode to measure serum Na concentration will avoid this problem since it is not affected by protein or lipid content. To maintain electrical neutrality, Cl typically moves either with Na or opposite to HCO3. The factors that lead to hypernatremia and hyponatremia similarly affect Cl levels. To determine if the cause of hypochloremia or hyperchloremia is due to the same process affecting Na concentration, one can perform an easy calculation to “correct” Cl for the concentration of Na. This is done by dividing “normal” Na (typically a value from the middle of the reference interval) by the actual Na concentration and multiplying this result by the actual Cl concentration:
7
Acid–Base and Electrolytes
7.1 Acid–Base
7.1.1 Steps to Evaluating Acid–Base Status
7.1.2 Bicarbonate
7.1.3 Metabolic Acidosis and Alkalosis
7.1.3.1 Chloride‐Associated Acid–Base Abnormalities
Acid–base abnormality
pH
pCO2
HCO3
Cl
AG
High anion gap metabolic acidosis
with respiratory compensation
L
L
L
N
H
Hyperchloremic metabolic acidosis
with respiratory compensation
L
L
L
H
N
Hypochloremic metabolic alkalosis
with respiratory compensation
H
H
H
L
N
Respiratory acidosis
with metabolic compensation
L
H
H
L
N
Respiratory alkalosis
with metabolic compensation
H
L
L
H
N
7.1.3.2 Anion Gap
7.1.4 Respiratory Acidosis and Alkalosis
7.1.5 Compensatory Mechanisms
7.1.6 Base Excess
7.2 Electrolytes
7.2.1 Sodium
7.2.1.1 Hypernatremia
Sodium
Hypernatremia
Dehydration
Insensible water loss
Diabetes insipidus (central or nephrogenic)
Hyponatremia
Hypertonic fluid loss or isotonic loss with water repletion
Gastrointestinal disease
Renal disease
Sweat loss
Electrolyte and fluid shifts due to third‐spacing of fluids
Uroperitoneum
Severe hypoalbuminemia
Severe hepatic disease
Severe cardiac disease
Chloride
Hyperchloremia
See hypernatremia
Metabolic acidosis
HCO3 loss or sequestration
Intestinal disease
Renal loss
Metabolic compensation for respiratory alkalosis
Hypochloremia
See hyponatremia
Metabolic alkalosis
HCO3 retention with Cl loss/sequestration
Upper gastrointestinal obstruction
Sweat loss
Metabolic compensation for respiratory acidosis
7.2.1.2 Hyponatremia
7.2.2 Chloride
7.2.2.1 Hyperchloremia and Hypochloremia with Water Imbalance
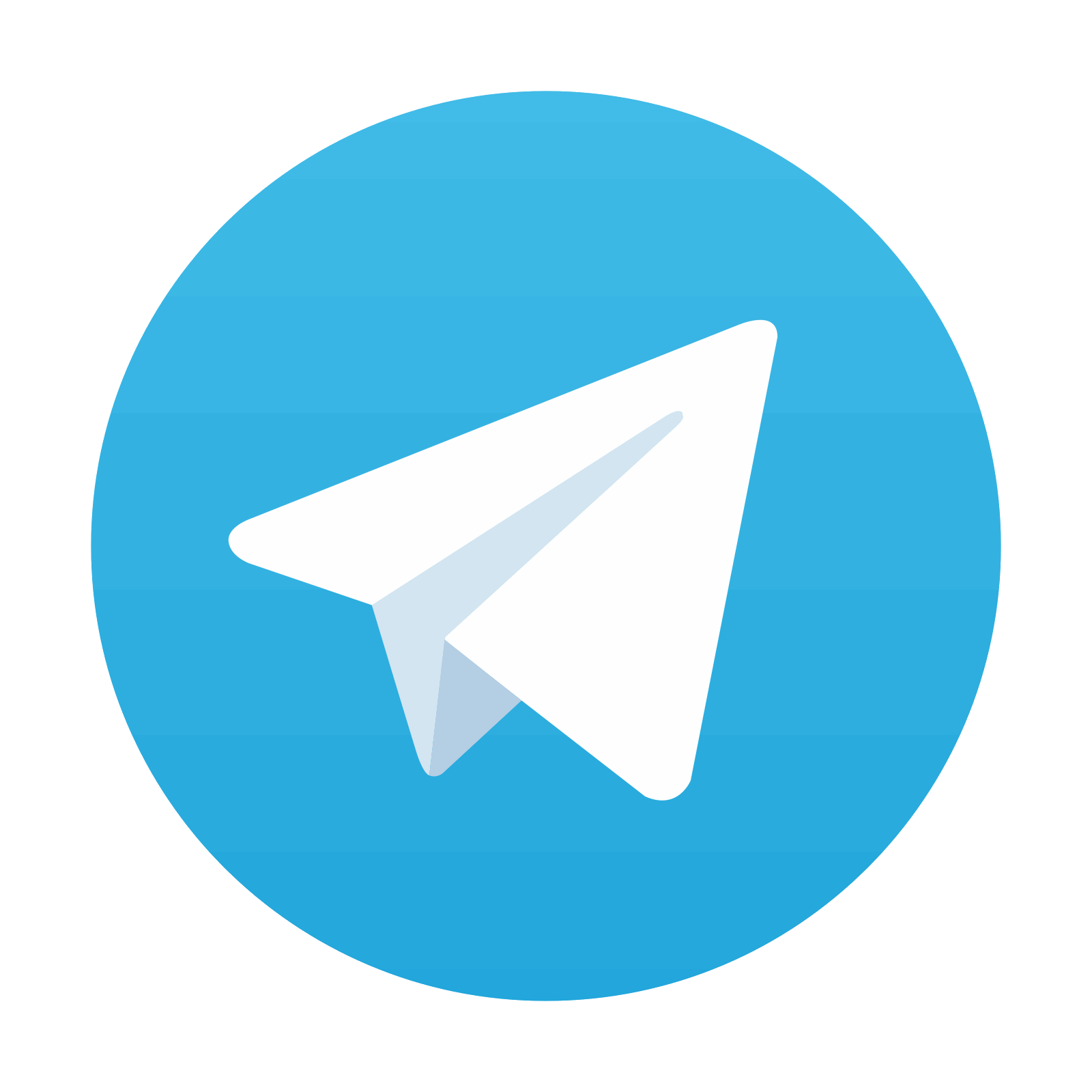
Stay updated, free articles. Join our Telegram channel

Full access? Get Clinical Tree
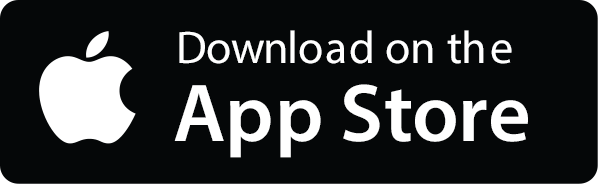
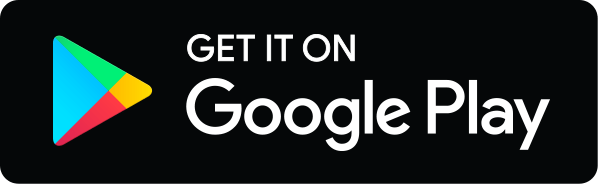