Nathan C. Nelson Clinical Professor, Diagnostic Imaging, Department of Molecular Biomedical Sciences, College of Veterinary Medicine, North Carolina State University, Raleigh, NC, USA Osseous anatomy is well suited for radiographic evaluation. The high mineral content within bones imbues them with improved x‐ray attenuation ability compared to surrounding soft tissue structures. This differential attenuation results in high radiographic contrast between osseous and soft tissue structures. Pathology that alters bone density or bone margination is therefore readily recognized. For that reason, radiographs are typically the initial screening test when musculoskeletal disease is suspected. While osseous structures are relatively easy to identify, radiographs are much more limited in their ability to evaluate the soft tissue components of the musculoskeletal system. Given their similar density and constitution, muscles, tendons, ligaments, and vascular structures share the same opacity. Where these soft tissues contact one another, no distinct margin is identified. This so‐called “silhouette sign” often prevents more exact determination of the nature of the soft tissue disease, instead resulting in a generalized, nonspecific swelling on radiographs. Direct imaging of soft tissue musculoskeletal structures requires advanced imaging. Ultrasound in particular is well suited for evaluation of peripheral/appendicular soft tissue pathology as it readily discriminates between types of soft tissues (tendon, ligament, muscle, vessel, connective tissue). The superficial nature of the peripheral limb soft tissues makes them readily accessible to ultrasound evaluation. Despite its limited ability to evaluate soft tissues, radiography remains the mainstay of musculoskeletal imaging. For that reason, chapters on musculoskeletal radiography will focus on primarily on this modality. Advanced imaging with CT, MRI, and ultrasound is growing in use, and in some applications is preferred to radiography, so will be discussed where these modalities are most commonly used. The skull and spinal column are particularly complex structures, with intricate osseous anatomy (such as the vertebrae) surrounding soft tissue structures (such as the spinal cord), so the chapters on imaging of the head and spine will naturally include more MRI and CT images than in other areas of the body. As discussed above, the appendicular skeleton is more readily amenable to ultrasound imaging and so normal ultrasound anatomy of this area will be included in the relevant chapter. This introductory chapter will outline the basic principles of musculoskeletal imaging, including choice of modality, patient positioning, and a review of normal anatomy (see also Appendix I). Clinically relevant anatomic variants are included and should not be misidentified as clinically significant pathology. As with other areas of the body, appendicular radiography requires a minimum of two orthogonal projections. Single radiographs can result in failure to recognize clinically significant abnormalities (Figure 6.1). The complexity of the skeleton dictates a wider variety of additional supplementary projections compared to the thorax or abdomen. Which projections constitute a “complete study” depends on the anatomic region, clinician preference, and the clinical question to answer. As an example, the author considers four projections a minimum study for the carpus and tarsus, as two projections are frequently insufficient to fully characterize the complex anatomy of these areas. Table 6.1 lists body regions with recommended projections that constitute a “complete radiographic study” (and includes figure references). Optional projections and their indications are also listed. FIGURE 6.1 Ventrodorsal (A) and lateral (B) projections of the pelvis demonstrating the importance of orthogonal projections. On the ventrodorsal projection, the ball of the left total hip replacement appears to be superimposed with the acetabulum, however on lateral projections it is dorsally luxated (arrow). TABLE 6.1 List of appendicular regions, with projections that constitute a complete baseline study and optional additional projections. Complete radiographic projections are included (and colloquial/abbreviated names for those projections are in parentheses). FIGURE 6.2 Standard radiographs of the carpus consisting of lateromedial (A), dorsopalmar (B), dorsal 45 lateral‐palmaromedial oblique (C), and dorsal 45 medial‐palmarolateral oblique (D) images. FIGURE 6.3 Standard radiographs of the tarsus consisting of lateromedial (A), dorsoplantar (B), dorsal 45 lateral‐plantaromedial oblique (C), and dorsal 45 medial‐plantaromedial oblique (D) images. FIGURE 6.4 Standard radiographs of the manus consisting of lateromedial (A), dorsopalmar (B), dorsal 45 lateral‐palmaromedial oblique (C), and dorsal 45 medial‐palmarolateral oblique (D) images. Notice that similar to carpus projections (Figure 6.2), the entire carpus is included on these projections, but unlike carpal projections the projection is not centered on the carpus and includes the entire digits. FIGURE 6.5 Lateromedial splay toe projection of the manus, which allows evaluation of the digits within the superimposition present on a nonsplay toe lateromedial projection. This projection is acquired by placing gauze or tape around the nail of the first and fourth digits and displacing one dorsally and one palmarly. FIGURE 6.6 Lateromedial (A) and craniocaudal (B) projections of the elbow. FIGURE 6.7 Flexed lateromedial projection of the elbow, acquired when evaluation of the anconeal process (arrowhead) is necessary. FIGURE 6.8 Cranial 15° medial‐caudolateral oblique projection of the elbow to better evaluate the medial coronoid process. By positioning the elbow in this manner, the medial coronoid process extends more medially without superimposition of the rest of the ulna, allowing visualization of more of it compared to the standard craniocaudal projection. FIGURE 6.9 Normal lateral (A) and craniocaudal (B) projections of the shoulder. FIGURE 6.10 Normal cranioproximal to craniodistal oblique of the shoulder, usually referred to as the shoulder skyline projection, used to see the greater tubercle and the intertubercular groove (arrowhead). Notice the smooth margins of the groove. FIGURE 6.11 Normal ventrodorsal (A) and lateral (B) projection of the pelvis. Note that the ventrodorsal projection includes the entire femurs. FIGURE 6.12 Frog‐leg ventrodorsal projection of the pelvis, used to better evaluate the femoral heads. FIGURE 6.13 Distraction view of the pelvis (A) compared to a standard ventrodorsal projection (B) in a normal dog. A PennHip distractor is used (margins of the distractor are indicated by arrowheads) to distract the hips. The coxofemoral joints are widened on the distracted view compared to the standard projection. FIGURE 6.14 Dorsal acetabular projection of the pelvis in a normal dog. This projection is used to evaluate the dorsal acetabulum margin (arrowheads) for osteophytes or fractures or other pathology. Normally the acetabular rim is superimposed with the femoral heads, preventing their evaluation on ventrodorsal projections. FIGURE 6.15 Lateromedial and craniocaudal projection of the scapula in a normal dog. Note that on the lateral projection, there is superimposition of the scapula with the spine. FIGURE 6.16 Caudocranial (A), neutral lateral (B), and extended lateral (C) projections of the stifle in a normal dog. The neutral positioning (with the femur and tibia approximately 90° to one another) is the preferred position for radiographing the stifle prior to a TPLO procedure. In comparison, the stifle is extended when radiographs are performed prior to a TTA procedure. This positioning results in an angle closer to what a dog would have during normal standing (approximately 135° angle between the femur and tibia). As a reminder, radiographic projections are named for the direction of the x‐ray beam; the region of entry and region of exit are listed in that order. If obliques are performed, the angle of obliquity relative to beam entry is listed. Table 6.1 lists projections with complete and colloquial names; a detailed description of radiographic naming conventions is available, though often shortened to a colloquial or abbreviated name for everyday use [1]. Proper positioning of musculoskeletal radiographs is critical. Even slight obliquity can hide clinically significant pathology (Figure 6.17). Poor positioning can give the false impression of pathology when none is present (Figure 6.18). Poor positioning also distorts normal anatomy (Figure 6.19). FIGURE 6.17 Lateromedial projections of a well‐positioned (A) and poorly positioned spine (B), with magnified C3–4 disc spaces of the well‐positioned (C) and poorly positioned (D) images. On the well‐positioned spine (A,C), intervertebral disc spaces are clearly identified without overlap of adjacent vertebral bodies. There is superimposition of the left and right transverse processes. On the poorly positioned spine (B,D), there is overlap of adjacent vertebral bodies (open arrowhead) and one set of transverse processes is more ventral than the others due to rotation along the long axis of the spine (closed arrowheads). FIGURE 6.18 Ventrodorsal projections of the pelvis with the legs slightly abducted compared to a properly positioned projection with the legs more adducted. Notice with proper positioning the patellas are superimposed with the distal femur (arrowheads) as opposed to the poorly positioned projection where they are laterally located (arrows). This poor positioning results in the false impression of lateral patellar luxation. FIGURE 6.19 Ventrodorsal projections with the legs incompletely extended (A), resulting in foreshortening compared to the better positioned pelvis with femurs more extended (B). Ultrasound is increasingly used in appendicular imaging as a quick, relatively inexpensive test to diagnose and monitor a variety of soft tissue injuries. Peripheral tendon disease is easily accessed with this modality, and new high‐resolution linear transducers provide the detail necessary to identify the tendon ultrastructure for evidence of disruption. Biceps tenosynovitis and common calcaneal tendonitis or disruption are the most common diseases diagnosed with ultrasound, though it is suitable for examination of any soft tissues not surrounded by bone. Computed tomography (CT) use in small animal appendicular imaging is more limited. Its primary use is evaluation of elbow dysplasia as radiographs may not be able to diagnose the subtle pathology present in early disease or may underrepresent the severity of disease even if changes are present. Given its ability to recreate complex three‐dimensional structures, CT is useful for preoperative planning in patients with complex angular limb deformities. CT also sees use in evaluation of potentially aggressive peripheral bone lesions, as it is more sensitive to evidence of inflammation and bone neoplasia compared to radiographs. Magnetic resonance imaging (MRI) evaluation of appendicular limb musculoskeletal pathology is rare. The high cost is prohibitive for many clients. Compared to humans, the small size of canine/feline joints, tendons, ligaments, and cartilage poses a challenge, as many MRI sequences cannot provide the high resolution necessary to image such small structures within a reasonable amount of time. Moreover, for most common canine and feline appendicular pathology, clinical examination paired with radiographs provides a definitive diagnosis without the need for an expensive imaging test. As an example, palpation of a cranial drawer sign in a patient with suspected cranial cruciate ligament pathology is diagnostic for that disease, and surgical stabilization methods do not depend on advanced preoperative imaging beyond radiographs. The tibia/fibula and radius/ulna are particularly affected in patients with chondrodystrophy (Figure 6.20). There is procurvatum and external rotation of the paws in these patients. No clinically significant elbow incongruity is expected. The femur in these breeds is typically short with larger peripheral features than other breeds. The humerus has a more curved proximal diaphysis, with a hook on the caudal aspect of the humeral head that is not an osteophyte. FIGURE 6.20 Lateromedial (A) and craniocaudal projection (B) of the thoracic limbs of a chondrodystrophic versus a nonchondrodystrophic dog (C,D). Notice in the chondrodystrophic dog there is cranial curvature of the middiaphysis of the radius (procurvatum) and ulna, with valgus and rotation of the manus centered at the carpus. Smaller sesamoid bones should not be confused with pathology (such as chip fragments). The supinator sesamoid bone is adjacent to the radial head, but is variable between patients, sometimes not seen at all, and in some patients seen unilaterally or bilaterally (Figure 6.21). The abductor pollicus longus sesamoid bone is consistent in dogs, seen medial to the carpus (Figure 6.22). The sesamoid bones palmar to the metacarpo/tarsophalangeal joints are always present (though the first digit only has one) and the dorsal sesamoid at these joints is also consistently identified. The popliteal sesamoid and fabellae (sesamoids of the gastrocnemius muscle) are common and well‐understood sesamoid bones. FIGURE 6.21 Normal‐appearing supinator sesamoid bone adjacent to the radial head (arrow). FIGURE 6.22 Dorsopalmar (A) and dorsolateral‐palmaromedial oblique (B) radiographs of a normal‐appearing abductor pollicis longus sesamoid bone medial to the carpus (arrowheads). A focal, small mineralized body caudal to the glenoid cavity in a dog shoulder is an ossification variant and not associated with other degenerative change (Figure 6.23). Similarly, a small secondary center of ossification of the cranial rim of the acetabulum in juvenile animals typically fuses by 1 year of age (Figure 6.24). A focal mineralized body may also be seen proximal to the greater trochanter, at the site of insertion of the gluteal musculature (Figure 6.25). This is not associated with clinical signs. FIGURE 6.23 Lateromedial radiograph of the shoulder showing a small ossification center (arrow) caudal to the scapula. FIGURE 6.24 Ventrodorsal projection of the pelvis showing small secondary centers of ossification cranial to both acetabula (arrows). FIGURE 6.25 Ventrodorsal projection of the pelvis showing focal rounded mineralization proximal to both greater trochanters at the site of insertion of the gluteal musculature. This is not a clinically significant finding. Dog clavicles are small and inconsistently mineralized on radiographs, though some patients have large prominent clavicles (Figure 6.26
CHAPTER 6
Anatomy, Variants, and InterpretationParadigm
Overview
Appendicular Skeleton
Appendicular Skeleton: Basic Radiography Principles and Anatomy
Body part
Standard projections
Additional projections
Indication for projection
Carpus
Lateromedial (lateral; Figure 6.2a)
Dorsopalmar (DP; Figure 6.2b)
Dorsal 45° medial‐palmarolateral oblique (45° medial oblique; Figure 6.4c)
Dorsal 45° lateral‐palmaromedial oblique (45° lateral oblique; Figure 6.3d)
Extended lateromedial
Dorsopalmar with medial stress
Dorsopalmar with lateral stress
Palmar instability
Medial instability
Lateral instability
Tarsus
Lateromedial (lateral; Figure 6.3a)
Plantarodorsal (PD; Figure 6.3b)
Dorsal 45° lateral‐plantaromedial oblique (45° lateral oblique; Figure 6.3c)
Dorsal 45° medial‐plantarolateral oblique (45° medial oblique; Figure 6.4d).
PD with medial stress (Chapter 8; Figure 8.32)
PD with lateral stress
Medial instability
Lateral instability
Pes/manus
Lateromedial (lateral; Figure 6.4a)
Dorsopalmar/plantar (DP; Figure 6.4b)
Dorsal 45° lateral‐palmaro‐(plantaro‐)‐medial oblique (45° lateral; Figure 6.4c)
Dorsal 45 medial‐palmaro‐(plantaro‐)‐lateral oblique (45° lateral; Figure 6.5d)
Splay toe lateral (Figure 6.5)
Better identification of distal phalanx from a lateral perspective without superimposition of the other phalanges
Elbow
Lateromedial (lateral; Figure 6.6a)
Craniocaudal (Figure 6.6b)
Flexed lateromedial (flexed lateral; Figure 6.7)
Cranial 15° medial‐caudolateral oblique (15° oblique; Figure 6.8)
Better identify osteophytes on anconeal process, without superimposition of the humerus
Better identification of the medial coronoid process and medial humeral condyle pathology
Shoulder
Lateromedial (lateral; Figure 6.9a)
Craniocaudal (Figure 6.9b)
Cranioproximal to craniodistal oblique (shoulder skyline; Figure 6.10)
Identify intertubercular pathology in cases with biceps tenosynovitis
Pelvis
Ventrodorsal with hips extended (VD pelvis; Figure 6.11a)
Lateromedial (lateral; Figure 6.11b)
Ventrodorsal with limbs abducted (frog‐leg projection; Figure 6.12)
Ventrodorsal with hip distraction (hip distraction view; Figure 6.13)
Dorsal acetabular rim projection (DAR projection; Figure 6.14)
Better evaluates femoral neck pathology such as capital physeal fractures by allowing the femoral head and neck to malalign
PennHip procedure used to screen for hip dysplasia (see Chapter 7)
Identifies early osteophyte formation on the acetabular rim, an area usually obscured by superimposition with the femoral head
Scapula
Lateromedial (lateral; Figure 6.15a)
Craniocaudal
(Figure 6.15b)
Stifle
Caudocranial (Figure 6.16a)
Lateral with a neutral position (lateral; Figure 6.16b)
OR
Lateral with an extended position (lateral; Figure 6.16c)
Prior to a tibial plateau leveling osteotomy (TPLO) procedure
Prior to tibial tuberosity advancement (TTA) procedure
Appendicular Skeleton: Advanced Imaging
Appendicular Skeleton: Anatomic Variants or Easily Confused Anatomy
Chondrodystrophy
Sesamoid Bones
Other Focal Mineralized Periarticular Bodies
Clavicles
Stay updated, free articles. Join our Telegram channel

Full access? Get Clinical Tree
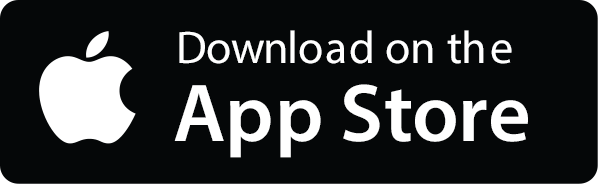
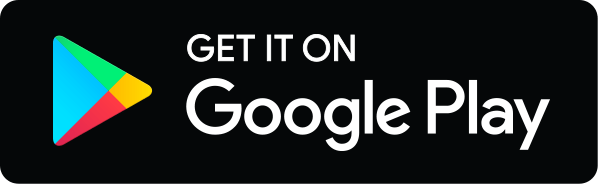