CHAPTER 5 Natara Loose Brooklyn, New York Glucose is required for energy production by every cell of the body, making it vital to the ongoing function and repair of all cells. Life‐sustaining activities such as heart rate and rhythm, vasomotor tone, respiration, and neurological and immune functions depend on having glucose available to the cells. In addition to energy production, glucose makes an important contribution to serum osmolarity, influencing fluid distribution throughout the body. Therefore, every patient in the intensive care unit is at risk for experiencing life‐threatening complications associated with alterations in blood glucose. The reported normal range for blood glucose is 67–168 mg/dL (3.71–9.32 mmol/L) in cats and 62–172 mg/dL (3.44–9.55 mmol/L) in dogs [1]. Box 5.1 provides conversion factors for changing mg/dL to mmol/L for glucose. Hypoglycemia is defined as a blood glucose concentration of <60 mg/dL (<3.33 mmol/L) for both species [2]. The definition of hyperglycemia varies between dogs and cats. For dogs, values greater than 120 mg/dL (6.66 mmol/L) indicate hyperglycemia [3]. Stress hyperglycemia has been shown to cause values as high as 613 mg/dL (34 mmol/L) in cats [4]. In general, fasting levels >140 mg/dL (7.77 mmol/L) should be considered as hyperglycemia in both the dog and cat. Following ingestion of a meal, glucose is extracted from the blood by the liver, brain, skeletal muscle, red blood cells, fat cells, and skin. The endocrine and autonomic nervous systems control moment‐to‐moment regulation of blood glucose [5]. The hormones responsible for endogenous glucose production are numerous and include insulin, glucagon, cortisol, and catecholamines (Table 5.1). Glucose is released on demand into the blood by the breakdown of endogenous glycogen stores, primarily by the liver. The pancreas responds to alterations in blood glucose by releasing either insulin or glucagon which signal the liver to either release or to store glucose, respectively (Figure 5.1). Glycogenolysis and gluconeogenesis contribute equally to the basal rate of hepatic glucose production. When insulin concentrations are low, hepatic glucose output rises. Table 5.1 Hormones that regulate blood glucose. Figure 5.1 The endocrine response to low and high blood glucose. Green arrows, mechanisms to reduce blood glucose: sites of glucose uptake and storage by cells after insulin release; insulin not required for uptake by liver and brain; insulin promotes glucose storage as glycogen in the liver; red arrow, inhibition of activity; blue lines, mechanisms to increase blood glucose. Since glucose cannot readily diffuse through most cell membranes, assistance from facilitated glucose transporter (GLUT) molecules and insulin is required for entry. The glucose transporters form an aqueous pore through which glucose can move into the cell more easily. The rate of glucose entry at these sites is proportional to the blood glucose levels. Glucose enters hepatic and pancreatic beta‐cells by GLUT‐facilitated diffusion and does not require insulin at these sites. Once within the cell, the glucose is phosphorylated and trapped. While the liver does not require insulin to facilitate glucose uptake, it does need insulin to regulate glucose output [6]. Within the pancreatic beta‐cells, glycolysis results in ATP production, providing the energy to release insulin into the blood by exocytosis. Insulin then reduces blood glucose levels by accelerating transport of glucose into insulin‐sensitive cells, facilitating glucose conversion to glycogen, promoting fat formation, and suppressing endogenous (primarily hepatic) glucose production. The majority of glucose uptake in peripheral tissue occurs in muscle, where it is used immediately for energy or stored as glycogen [7]. Adipose tissue contributes significantly to glucose homeostasis by regulating the release of free fatty acids that increase gluconeogenesis [8]. The kidneys play an important role in glucose homeostasis through gluconeogenesis and eliminating excess glucose in the urine [9]. The main consumer of glucose is the brain, accounting for approximately 50% of glucose use in the fasting phase [8]. Brain cells have high metabolic activity with neurons needing energy for depolarization, axonal and dendritic transport, and structural renovation of brain tissue [10]. Glucose enters the brain tissue by GLUT‐facilitated diffusion. The brain’s energy metabolism depends almost exclusively on the constant supply of glucose. The glucose supply required for normal brain function remains steady even during blood flow fluctuations [11]. Hypoglycemia stimulates the pancreatic alpha‐cells to produce glucagon (see Figure 5.1, Table 5.1). This hormone increases glucose levels by accelerating glycogenolysis and promoting gluconeogenesis. In response to the elevation in blood glucose, serum insulin levels rise, which then inhibit glucagon release and suppress hepatic glucose production. The complicated and critical role that glucose and the glucose regulatory hormones play in the function of every organ in the body makes blood glucose concentration one of the most important variables in critical illness. Discovering fluctuations in blood glucose can afford insight into the metabolic activity of the patient while providing direction for therapeutic intervention and minimizing complications. The evaluation of diagnostic and monitoring data will aid in the early detection of glucose abnormalities and in the detection of any potential underlying systemic disorder contributing to the illness. The patient history and physical parameters provide valuable information for assessing the urgency and frequency of blood glucose monitoring. The determination of blood glucose levels can be rapid and accurate by using cage‐side point of care (POC) testing. Further clinicopathological testing and diagnostic imaging may be necessary to identify an underlying cause. Potentially life‐threatening consequences of glucose alterations (such as seizures, tremors, coma) should be rapidly stabilized prior to initiating extensive diagnostic testing. The signalment (age, sex, breed), initial history, and physical examination are assessed for evidence of problems associated with alterations in blood glucose. Historical findings compatible with or suggestive of hypoglycemia and hyperglycemia can be found in Table 5.2. Young dogs and cats, toy breed dogs, and hunting dogs may have a propensity for hypoglycemia. Cats frequently experience transient hyperglycemia due to stress. The current diet and appetite of the patient can become significant when related to blood glucose. Past medical problems could reveal clinical signs of underlying problems that will manifest as an alteration in blood glucose. Exposure to drugs or toxins known to be associated with changes in blood glucose (Table 5.3) should be identified. Table 5.2 Common historical and physical findings with hyperglycemia and hypoglycemia in the dog and cat. Table 5.3 Drugs and toxins reported to affect blood glucose. This is a representation of agents and not intended to be a complete listing of all drugs and toxins affecting blood glucose. Clinical signs will be influenced by the degree and rapidity of onset of glucose alterations. The level of consciousness, cardiovascular status, muscle strength and tone, urine production, and state of hydration are of particular interest when evaluating the physical examination related to blood glucose. The body condition score may demonstrate cachexia or obesity, both related to alterations in blood gluocse. A list of physical signs common to acute and chronic glucose disorders is provided in Table 5.2. Hospitalized critical patients are to be examined at least twice daily for new, worsening or changing physical signs compatible with abnormalities in blood glucose. Typically, hypoglycemia is more consistent in showing overt clinical signs than hyperglycemia. Depressed mentation, tremors, seizures, and hypotension (weak or absent pulses, pale mucous membrane color) can be life‐threatening signs of acute and severe hypoglycemia. With chronic, gradual hypoglycemia, the clinical signs are often more vague. Foul‐ or sweet‐smelling breath (ketosis), depressed mentation or a plantigrade or palmigrade stance (Figure 5.2) can be signs of severe and chronic hyperglycemia. Figure 5.2 Plantigrade and palmigrade stance: clinical signs associated with chronic hyperglycemia. With therapy, this patient returned to normal neurological function and gait. The packed cell volume (PCV), total protein (TP), blood glucose, blood urea nitrogen (BUN), serum electrolytes, blood gas analysis, and coagulation profile (platelet estimate, prothrombin time, partial thromboplastin time) are considered crucial components of the minimum laboratory database for any critical animal. The PCV and TP can reflect anemia or hemoconcentration, each significant when assessing blood glucose results. A low BUN can direct investigation for liver disease, a known cause of hypoglycemia. The presence of a metabolic acidosis directs assessment of the electrolyte status and a search for blood and urine ketones. Hypokalemia and hypernatremia are important electrolyte changes that can occur with severe or chronic hyperglycemia. Hyperkalemia with hyponatremia can be a result of hypoadrenocorticism, a cause of hypoglycemia. Alterations in coagulation must be identified and can occur secondary to diseases such as hyperadrenocorticism, hypothyroidism, and renal disease, known to be associated with glucose disorders. Blood glucose testing becomes a priority for any patient displaying signs of severe hypoglycemia, such as ataxia, collapse, altered consciousness, depressed mentation, seizures, muscle tremors or twitching, and coma. However, any patient admitted to the hospital should have at least one blood glucose test performed daily. Additional measurements are indicated for those patients with identified glucose disorders, on dextrose supplementation, receiving parenteral nutrition or requiring insulin therapy. More frequent or continuous monitoring may be indicated depending on the underlying disease and patient physical condition. A single blood glucose measurement may not be an accurate representation of the blood glucose status over time. Therefore, the interpretation of any single result should be made in light of how the condition of the patient has changed over time. All hospitals should have a rapid, in‐hospital method for quantitating blood glucose. While visual assessment of glucose test strips provides a quick estimation, POC testing is more accurate and cost‐effective with portable blood glucose meters (PBGMs); the accuracy of the results from these POC glucose monitors has been investigated [12]. It is important to employ the manufacturer’s guidelines, proper instrument care, and appropriate sample collection and handling. User error has been cited as one of the most common reasons for inaccurate results from PBGMs. Human glucometers have consistently underestimated actual glucose concentrations in the blood of dog and cats [13]. The human glucometers rely on universal distribution of glucose between plasma to whole blood. Unfortunately, that glucose distribution in whole blood is more variable in dogs and cats [13]. Veterinary‐specific PBGMs are commercially available which have been tested and approved for analyzing canine and feline whole‐blood glucose from miniscule quantities of blood. Test results are immediate, with many models showing results in <6 seconds. The use, accuracy, and efficacy have been reported for the AlphaTRAK® (Abbott Laboratories, Abbott Park, IL) and GlucoPet® (Animal Diabetes Management, Janesville, WI) veterinary PBGMs [14–18]. The GlucoPet requires 1 μL for testing and the AlphaTRAK only 0.3 µ for accurate results. The g‐pet® (Woodley Equipment Copany, Horwich, UK), i‐pet® (UltiCare Inc., St Paul, MN), and GlucoPet have been shown to have comparable results to the AlphaTRAK [14–18]. The use of a lancet minimizes the volume of blood drawn and reduces the risk of anemia from repeated blood collection in smaller patients. The use of the marginal ear vein technique (Figure 5.3) has been shown to be effective and safe, and to provide blood glucose values comparable to a peripheral vein in healthy cats and cats with diabetes mellitus [19]. Figure 5.3 (a) A lancet can be used on the tragus of the ear to collect a drop of blood from the cat. (b) The lateral ear vein provides an additional source for collecting a drop of blood by lancet in the cat. The results from whole‐blood testing by PBGMs can differ from serum biochemistry blood glucose results, depending upon the patient hematocrit. A false elevation in blood glucose can be reported in dogs with anemia and a false decline in blood glucose in dogs with elevated packed cell volumes [20,21] using veterinary PBGMs. Results from PBGMs should be assessed in light of the patient hematocrit to avoid missing a true hypoglycemia in an anemic patient. Point of care measurement of blood beta‐hydroxybutyrate is available to diagnose ketonemia. The POC Precision Xtra® ketone meter (Abbott, Alameda, CA) was found to be a valid tool for the measurement of beta‐hydroxybutyrate in diabetic cats, with values <2.55 mmol/L excluding ketoacidosis [22]. Continuous glucose monitoring (CGM) is possible with interstitial glucose monitors (Figure 5.4). The CGMs measure the concentration of glucose in the interstitial space. Real‐time continuous glucose evaluation can provide important data in critically ill animals [23,24]. Figure 5.4 Continuous interstitial blood glucose monitoring system. (a) The sensor (needle) with transmitter. (b) The sensor and transmitter attached to the patient. (c) The real‐time interstitial glucometer with graphic (glucose curve) display. Information from the system can be wirelessly transmitted to the receiver to collect and download data. Most systems require several calibration points, often every 12 hours. Source: photos courtesy of Dr Tyler Klose (a, b) and Medtronic (c). Evaluation of CGMs in multiple veterinary populations has found the results to correlate well with serum reference laboratory results. These devices have been shown to provide early detection of hypoglycemia and demonstrate more accurate glucose trends over the course of treatment in diabetic animals compared to intermittent PBGM collection [25,26]. The use of CGMs in the ICU can also reduce the need for repeated blood collection, thereby improving patient comfort and potentially decreasing morbidity and mortality through prompt recognition and resolution of hyperglycemia or hypoglycemia. The CGM devices consist of a portable recording device and a flexible glucose sensor electrode. Once placed in the subcutaneous tissues, glucose is measured at set intervals, which may be as little as every 10 seconds. An average value is generated and recorded at regular intervals (such as every 5 minutes). Studies in the cat have shown that dorsal neck placement of the probe may be superior to lateral chest wall and lateral knee fold placement for accuracy of glucose measurement [27]. The electrodes have a single patient use and must be replaced at recommended intervals. Calibration of the unit, which requires collecting whole‐blood glucose, is required at least once during each 24‐hour period. As the technology advances, veterinary‐specific models with real‐time measurements may become available. The complete blood count (CBC), serum biochemical profile and urinalysis are important to identify disorders known to be associated with alterations in blood glucose. Neutrophilia with a left shift or leukopenia may direct diagnostic efforts to sepsis as a cause of hypoglycemia. A stress leukogram (neutrophila, eosinopenia, lymphopenia) may be consistent with a stress hyperglycemia. The serum biochemical profile is used to assess for potential underlying metabolic conditions that can be associated with glucose disorders. The concentration of liver enzymes, albumin, cholesterol, and glucose can suggest the presence of liver disease, a cause of hypoglycemia. An elevated alkaline phosphatase is often present in hyperadrenocorticism, known to be associated with hyperglycemia. The BUN and creatinine are evaluated as a reflection of renal function, since the kidney is important in glucose homeostasis. Pancreatitis is recognized as a cause of diabetes mellitus with elevated pancreatic enzymes supporting the diagnosis, though additional testing and imaging are warranted. The urinalysis can provide a significant amount of information in patients with glucose disorders. The urine specific gravity reflects renal function, with concentrated urine anticipated with dehydration from glycosuria in a normal kidney. Urine biochemical tests will detect the presence of glycosuria, ketonuria, proteinuria, and inflammatory cells, each a potential consequence of hyperglycemia. Urinary tract infection is a common consequence of glycosuria and can be diagnosed by microscopic examination of urine sediment for white blood cells and bacteria and urine culture. Additional clinicopathological testing is often required to definitely diagnose underlying disorders causing alterations in blood glucose. Evaluation of the resting cortisol and adrenocorticotropic hormone (ACTH) stimulation may reveal hyper‐ or hypoadrenocortical disease. A diagnosis of pancreatitis requires assessment of pancreatic lipase immunoreactivity and abdominal imaging. Fasting and postprandial bile acids and blood ammonia levels might be indicated to identify hepatic contributions to a glucose disorder. The assessment of plasma insulin concentrations compared to plasma glucose will aid in the diagnosis of insulinoma. A number of ratios have been utilized to aid in the diagnosis of an insulinoma (e.g. insulin:glucose ratio, amended insulin:glucose ratio). The most commonly utilized index is the amended insulin:glucose ratio which is calculated by the following equation: The insulin:glucose ratio must be assessed in conjunction with the historical and physical findings [28]. Fructosamine and glycosylated hemoglobin are two glycated proteins which can be quantitated in the serum for monitoring diabetic dogs and cats [29,30]. The two proteins reflect the mean blood glucose over a period of time. The levels are proportional to the blood glucose concentration and are not affected by stress. The bonding of glucose to proteins produces fructosamines. A single fructosamine measurement indicates the average glucose concentration over the previous 2–3 weeks. Single fructosamine measurements should be interpreted in light of clinical signs of diabetes, body weight, and blood glucose concentration. In general, the closer the fructosamine concentration is to the reference range for healthy dogs and cats, the better the glycemic control. When blood glucose levels are high, glucose molecules attach to the hemoglobin in red blood cells. The hemoglobin molecule is permanently glycosylated. The glycosylated hemoglobin level is proportional to the average blood glucose concentration over the previous four weeks to three months. Diagnostic imaging may be indicated to further characterize an underlying cause of a glucose disorder. Plain and contrast radiography can be used to demonstrate the size, shape, and location of internal organs and rapidly identify lesions suggestive of neoplasia. The presence of peritoneal or pleural effusions may be compatible with sepsis, a neoplastic process or liver failure, each potentially causing alterations in blood glucose. Studies have shown that the finding of a lower abdominal fluid glucose compared to the peripheral blood glucose is supportive of septic peritonitis (see Chapter 15). In addition to these findings, abdominal ultrasound can demonstrate less obvious changes in individual organ size and structure, neoplastic lesions, vasculature, and compartmentalized fluid. Ultrasound examination of the adrenal glands can identify adrenal tumors that cause hyperadrenocorticism and hyperglycemia or smaller adrenal glands, which can support the presence of hypoadrenocorticism causing hypoglycemia [31]. A skilled ultrasonographer may identify portovascular anomalies. When a definitive diagnosis of a persistent glucose disorder is not possible by plain radiographs or ultrasound, advanced imaging such as a portovenogram, nuclear scintigraphy, computed tomography, or nuclear magnetic imaging may be indicated. Variability in blood glucose levels during critical illness can have a deleterious impact on complications, length of hospital stay, and mortality in ICU patients. Acute hypoglycemia has been known to cause life‐threatening changes in brain function and vasomotor tone. Hyperglycemia affects fluid balance, brain function, and susceptibility to infection. The evaluation of blood glucose concentration by PBGMs enables rapid detection of glucose disorders. Therapeutic intervention for complications caused by hypoglycemia or hyperglycemia may be necessary prior to making the definitive diagnosis of the underlying disease state. Hypoglycemia presents the most immediate life‐threatening glucose disorder in the critical patient. It can be a direct result of the primary pathology, a consequence of the increased energy demands of critical illness or a side‐effect of medications administered (see Table 5.3). Symptoms attributable to hypoglycemia (see Table 5.2) in the critical patient are dependent upon the rate of change (acute or chronic) and the magnitude of change in blood glucose. Whipple’s triad has been used as a tool to make the definitive diagnosis of hypoglycemia when the diagnosis is uncertain and consists of (1) symptoms known to be caused by hypoglycemia, (2) low blood glucose at the time the symptoms occurred, and (3) reversal or improvement of symptoms or problems when blood glucose is returned to normal. When left untreated, hypoglycemia can result in permanent brain damage and eventually death. Neuroglycopenia causes dysfunction of cerebral Na‐K‐ATPase pumps and calcium channels. Subsequent cellular swelling and membrane disruption further augment neuronal excitotoxicity, leading to a series of events that risk permanent neuronal death [32]. The neurological symptoms of hypoglycemia could be overlooked due to similarity to preexisting signs of the underlying critical illness or the presence of sedation. Close and reliable monitoring of the glycemic level is crucial for rapidly detecting and treating hypoglycemia. A mild decline in blood glucose concentration (blood glucose between 65 and 80 mg/dL (3.6–4.4 mmol/L) causes inhibition of insulin secretion from the pancreatic beta‐cells. This results in decreased use of glucose by peripheral insulin‐dependent tissues and removal of the blockade on hepatic gluconeogenesis. Should the blood glucose fall below 65 mg/dL (3.57 mmol/L), glucagon secretion rises, contributing to an increase in blood glucose concentration. At this time the brain senses the drop in blood glucose and triggers a central sympathetic response (Figure 5.5). Metabolically, these compensatory mechanisms lead to increased glucose production through glycogenolysis initially and then gluconeogenesis, decreased muscle glucose oxidation and storage and increased release and use of alternate fuels, primarily free fatty acids. The free fatty acids are converted by the liver into ketone bodies, which can be used by the brain as an energy source. The restoration of plasma glucose to normoglycemia diminishes central sympathetic outflow. Figure 5.5 The effects of hypoglycemia on the brain and pancreas. Hypoglycemia stimulates an increase in the sympathetic response from the brain, resulting in increased hepatic glycogenolysis, muscle glycogen degradations, and lipolysis with the release of free fatty acids. The sympathetic‐mediated increase in blood flow to the pancreas stimulates the alpha‐cells to release glucagon and inhibits beta‐cell release of insulin. There is also direct suppression of the beta‐cells by the alpha‐adrenergic sympathetic response. α, alpha; β, beta; FFA, free fatty acids. Should hypoglycemia persist, growth hormone and cortisol release contribute to an increase in blood glucose. Cortisol enhances the mobilization of amino acids from proteins in many tissues and enhances the ability of the liver to convert these amino acids into glucose and glycogen by activating gluconeogenesis. The acute metabolic effects of growth hormone (see Table 5.1) oppose the normal effects of insulin on peripheral tissues and have been called the antiinsulin action of growth hormone [33]. In acute or chronic presentations, clinical signs are typically influenced by both the rate and magnitude of onset of hypoglycemia. Blood glucose values typically need to be <64.8–68.4 mg/dL (3.5–3.79 mmol/L) to cause any clinical signs specifically attributable to hypoglycemia [2]. Depressed mentation, tremors, seizures, and hypotension (weak or absent pulses, pale mucous membrane color) can be life‐threatening signs of acute and severe hypoglycemia. When hypoglycemia is acute, the patient is likely to manifest the classic neurological signs [10,34,35]. With chronic, gradual hypoglycemia, the clinical signs are often more vague. Table 5.2 lists the historical and physical examination findings typical of acute and chronic hypoglycemia. The etiology of hypoglycemia can be divided into three broad categories: (1) decreased glucose production, (2) increased glucose consumption, or (3) increased glucose loss. Common causes of hypoglycemia in the dog and cat are listed in Table 5.4, with a summary of the mechanism(s) causing the low glucose. In addition to the comments below, diagnostic and initial therapeutic interventions for common causes of hypoglycemia are presented in Table 5.5. Table 5.4 Differential diagnosis for causes of hypoglycemia in the dog and cat. Source: adapted from Koenig A. Hypoglycemia. In: Small Animal Critical Care Medicine, 2nd edn. Silverstein DC, Hopper K, eds. St Louis: Elsevier Saunders, 2015: pp 352–6. Table 5.5 Diagnotic testing and possible treatment plans for common causes of hypoglycemia in critical small animal patients. The initial goal of therapy is to supplement glucose to a level adequate to eliminate detrimental clinical signs. The method of treatment is guided by the severity of clinical signs and the suspected underlying cause of hypoglycemia. The presence of critical clinical signs such as dementia, seizures, coma, tremors, hypotension, and generalized weakness warrants immediate correction of hypoglycemia with intravenous (IV) glucose (also labeled as dextrose) supplementation. Intravenous or intraosseous access is required and 0.5 g/kg of dextrose as a 25% dextrose solution is administered. Persistent or refractory hypoglycemia may necessitate adding dextrose to the IV fluids. The goal is to administer the lowest concentration of dextrose that will maintain the blood glucose concentration between 80 and 100 mg/dL (4.44–5.55 mmol/L). Up to 7.5% dextrose can be given through a peripheral vein. When a higher concentration is required, central venous access is necessary to avoid phlebitis [37]. Since glucose will cause an intracellular shifting of serum potassium, monitoring blood potassium levels is necessary during glucose infusion. Potassium supplementation is provided in the IV crystalloids as outlined in Box 5.2 below. When a patient does not respond to glucose supplementation or requires increasing amounts of glucose for stabilization, investigation for causes such as insulinoma, septicemia or organ ischemia should be initiated.
Glucose
Introduction
Hormone
Site of production
Glucose‐related action
Effect on blood glucose
Insulin
Pancreatic beta‐cells
Enhances glucose entry into cells; storage of glucose as glycogen or fatty acids
Suppresses breakdown of proteins and fats to amino acids and free fatty acids (antilipolytic), suppresses insulin secretion
Regulates glucose release from hepatic cells
Glucagon
Pancreatic alpha‐cells
Release of glucose from glycogen; promotes glucose production from amino acids and fatty acids
Amylin
Pancreatic beta‐cells
Secreted with insulin; slows the rate of postprandial blood glucose appearance; promotes gastric emptying; promotes satiety
Somatostatin
Pancreatic delta‐cells
Local suppression of glucagon release from alpha‐cells; suppresses release of insulin, gastrin and secretin
Incretins
GIP
GLP‐1
Gastrointestinal tract
Postprandial: enhance insulin release and inhibit glucagon release; reduced gastric emptying to slow nutrient absorption
Epinephrine
Adrenal gland
Release of glucose from glycogen; release of fatty acids from fat cells
Cortisol
Adrenal gland
Antagonizes insulin; promotes gluconeogenesis
Adrenocorticotropic hormone
Anterior pituitary gland
Promotes release of cortisol and release of fatty acids from fat cells
Growth hormone
Anterior pituitary gland
Stimulation of lipolysis in fat; decreased glucose uptake by muscle; increased hepatic gluconeogenesis
Thyroxine
Thyroid gland
Release of glucose from glycogen; enhanced absorption of sugars from gastrointestinal tract
Monitoring methods
History and physical exam
Historical findings
Acute
physical findings
Chronic
physical findings
Hypoglycemia
Toy breed, decreased appetite, gastrointestinal signs, neurological changes, exposure to toxins, medications (insulin), history of liver disease, prolonged fever, prolonged tremors or seizures
Ataxia, weakness, tachyarrhythmias, bradyarrhythmias, hypertension, nonresponsive hypotension, altered mentation, tremors, muscle fasciculations, seizures, blindness, coma, death
Lethargy, altered appetite, weakness, paresis, hypotension, bradycardia
Hyperglycemia
Polyuria, polydipsia, polyphagia, severe stress
Dehydration, weight loss, polyuria, polydipsia, polyphagia, weakness, peripheral neuropathy, altered mentation, stupor, coma, death
Weight loss and muscle wasting, plantigrade stance, cataracts
Hyperglycemia
Hypoglycemia
Corticosteroids
Octreotide
Beta‐blockers
Epinephrine
Thiazide diuretics
Niacin
Pentamidine
Protease inhibitors
L‐asparaginase
Acute amphetamine ingestion
Zyprexa (olanzapine) and
Cymbalta (duloxetine)
Dexmedetomidine
Acetohexamide
Amprenavir
Chloramphenicol
Chlorpromazine
Chlorpropamide
Cibenzoline
Clove
Ethanol
Ethionamide
Fluorodeoxyglucose
Gatifloxacin
Ginseng
Glibenclamide
Gliclazide
Glimepiride
Glipizide
Gliquidone
Insulin
Insulin‐like growth factor
Pentamidine
Perazine
Pipothiazine
Quinine
Somatostatin
Sulfamethoxazole
Temafloxacin
Tolazamide
Tolbutamide
Trimethoprim
Xylitol
Minimum database
Point of care testing
Interstitial glucose monitors
Initial clinicopathological tests
Additional clinicopathological testing
Diagnostic imaging
Disorders of glucose
Hypoglycemia
Clinical signs
Disorder
Mechanism of hypoglycemia
Artifactual hypoglycemia
Improper handling of samples
Decreased glucose production
Hypoadrenocorticism
Cortisol deficiency
Liver disease, hepatoportal
Failure of gluconeogenesis, insufficient hepatic glycogen stores
Glycogen storage disease
Hepatic enzyme deficiency
Toy breed, puppy hypoglycemia
Alanine deficiency, limited hepatic glycogen stores, decreased muscle mass, lack of adipose tissue, impaired gluconeogenesis
Long‐term starvation
Decreased intake of components for glucose production
Increased glucose consumption
Sepsis
Hypoxic injury to hepatic cells, utilization of glucose by bacteria, increased tissue utilization of glucose, other mechanisms
Toxicosis
Xylitol, other medications (see Table 5.3)
Insulinoma
Insulin‐secreting pancreatic beta‐cells neoplasm
Other neoplasia
Insulin‐like factors, liver metastasis, glucose utilization by large tumors
Polycythemia, high white blood cell counts
Increased utilization by red blood cells or white blood cells
Insulin overdose
Hyperinsulinism
Increased glucose loss
Renal disease
Renal tubular problem causing glycosuria
Disease
Diagnostics
Treatment plan
Hypoadrenocorticism
Baseline cortisol
Dexamethasone NaP IV 0.1–0.3 mg/kg
ACTH stimulation test
DOCP 2 mg/kg (only if indicated)
Pre, 1 μg/kg cortisyn IV, 1 hour post
Prednisone 0.5–1 mg/kg/day PO
Hepatopathy
Ultrasound (hepatic size, vascular anomalies)
Surgical correction (portovenous anomaly)
Blood ammonia level Ammonia level
Nutritional support based on needsNutriceuticalsAntibiotic coverage
Bile acids (pre/post‐prandial)
Scintigraphy/MRI
Toxin
Thorough history
Activate charcoal depending on need
Supportive care
Neoplasia
Ultrasound
Surgical removal of neoplasia
Thoracic radiographs/CT scan
Chemotherapy
Amended Insulin:glucose ratio
Glucagon CRI (insulinoma)
Renal insult
Urinanalysis for glucosuria
Removal of nutritional influence if any
Ultrasound
Renal diet
Urine culture/susceptibility & MIC
Dietary history (Fanconi’s syndrome)
Septicemia
Complete blood count
Antibiotic coverage guided by diagnostics
Serum chemistry
Surgical intervention when appropriate
Abdominal ultrasound
Fluid supportive care (see Chapter 2)
Abdominal radiographs
Nutritional support (see Chapter 16)
Thoracic radiographs and/or CT scan
Urine culture/susceptibility & MIC
Blood culture/susceptibility & MIC
Fluid analysis
Blood pressure monitoring
Therapy
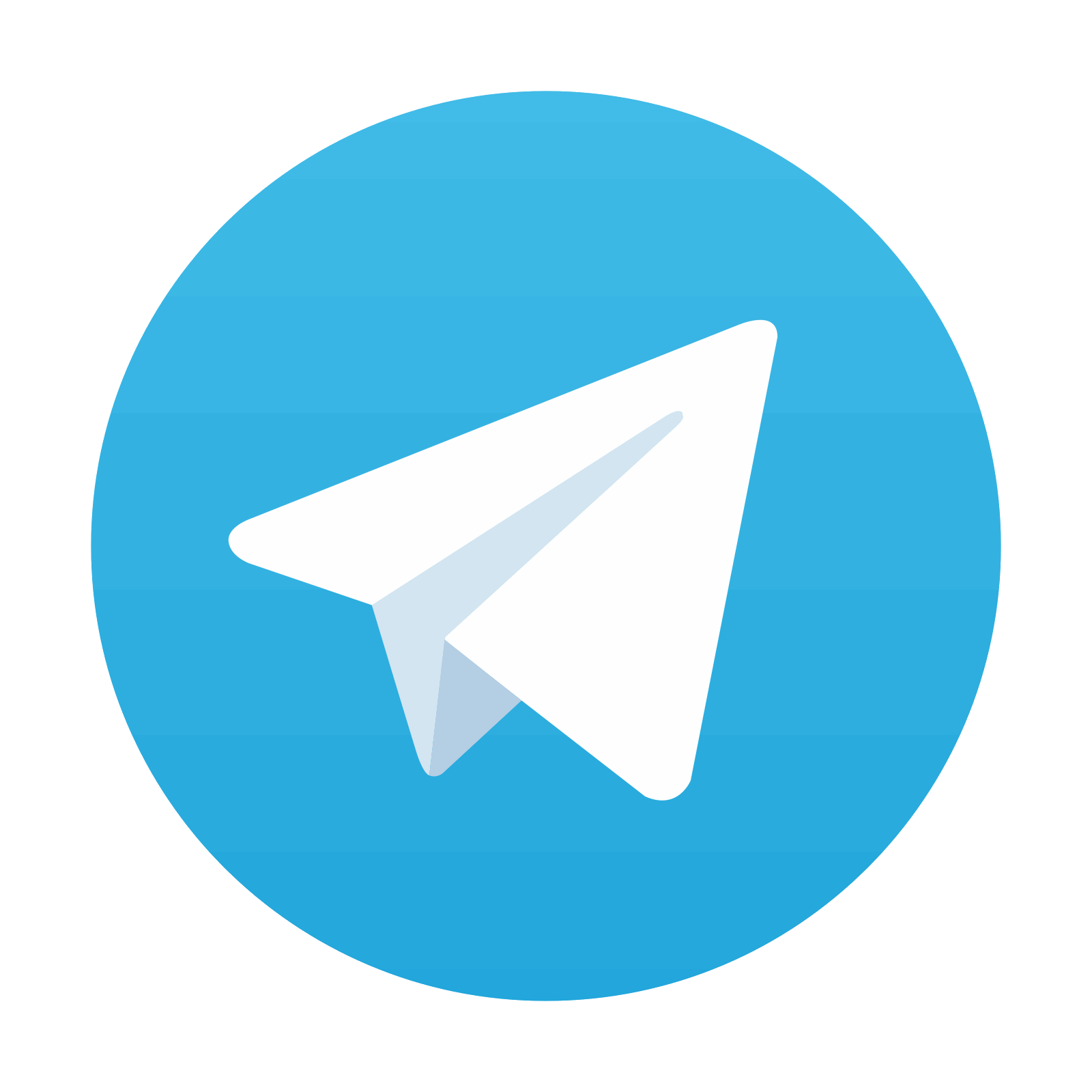
Stay updated, free articles. Join our Telegram channel

Full access? Get Clinical Tree
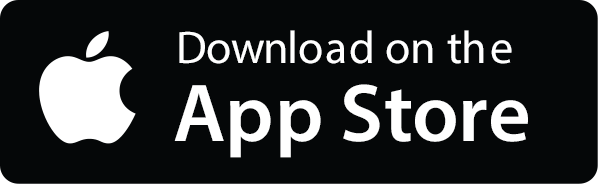
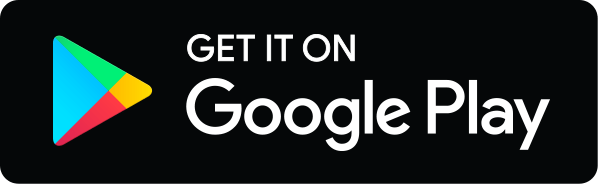