Paul Schwarzmann Tierklinik Schwarzmann, Rankweil, Austria The use of three‐dimensional (3D) printing, also referred to as “rapid prototyping” or “additive manufacturing,” in a medical setting began in the 1980s in human medicine.1 To the author’s best knowledge, the use of 3D‐printing in veterinary medicine was first described in 2003 for the assessment and preoperative planning for the treatment of bilateral pelvic limb deformities in a German Shepherd dog.2 Nowadays, 3D‐printing in veterinary surgery is used for several applications; while patient‐specific saw guides are mainly used for deformity correction of long bones,3–8 3D‐printed drill guides have various applications, such as the treatment of vertebral instability,9–18 humeral intracondylar fissure,19 ununited anconeal process (UAP),20 and other procedures. The basic concept of a 3D‐printed, patient‐specific surgical guide (3DP‐PSG) is mostly the same: a negative imprint of the bone surface is created, which sits “press‐fit” on the bone like a key in a keyhole. For instance, drill guides or saw guides direct either a drill or a saw in the preoperatively planned direction and thereby allow accurate execution of a surgical plan. In general, the production of a 3DP‐PSG consists of four steps: obtaining imaging data of the patient through computed tomography (CT) or magnetic resonance imaging (MRI),21 3D‐data acquisition from the obtained diagnostic imaging, guide design, and 3D‐printing. Since 3D‐printers are more affordable by now, a newer application of 3D‐printing in veterinary medicine is surgical education and training. The following chapter describes the development and process of a patient‐specific, 3D‐printed drill and saw guide, its use during surgery, the advantages and disadvantages of 3DP‐PSGs, and the use of 3D‐printing in surgical education and training. For the 3D‐planning of a specific procedure and the design of a 3DP‐PSG, it is necessary to obtain an accurate 3D model of the desired bone. It is possible to gain 3D‐data from CT or MRI although CT has a higher accuracy for bone models and is, therefore, recommended.22 For the CT scan, the desired bone should be positioned perpendicular to the CT gantry to avoid artifacts in the resulting 3D model. The slice thickness should be as thin as possible to get the highest resolution. Higher radiation settings in CT result in a higher accuracy of the resulting bone model.23 The imaging data is exported as Digital Imaging and Communications in Medicine (DICOM) file format.21 The process of obtaining a 3D bone model from DICOM images is called segmentation. Several commercial and free open‐source software programs are available to perform this task. Commonly used commercial software programs for segmentation of CT data are Mimics (Materialise, Leuven, Belgium),5,7,24,25 and OsiriX (Pixmeo, Bernex, Switzerland).6,8,14,15,19,26 A free, open‐source software commonly used for segmentation is 3D slicer (https://www.slicer.org).27,28 Other free, open‐source software used by the author is the software Horos (https://horosproject.org) and ITK‐SNAP (http://www.itksnap.org).20,29 The main differences between the mentioned software programs are the user friendliness, the tools available for segmentation, and the operating system it runs on. The decision of which software to use should be based on the needs, experience and personal preference of the user, the computing power and the operating system of the personal computer (PC), and the costs of the software. With most of these software programs, bones can be either automatically or manually segmented. For automatic segmentation, a region of interest (ROI) needs to be selected and certain structures can be isolated based on their gray value (threshold).30 The higher the threshold is set, the brighter the gray value of the pixels that are selected. Some software offers threshold presets for bone in CT scans, which may be sufficient in most cases but can possibly lead to insufficient accuracy of the resulting bone model since the gray value of bone can depend on the CT scanner, the ROI, and the patient itself.31 Therefore, the selection of the threshold value is a subjective setting and can have a high influence on the accuracy of the resulting bone model. A threshold value that is selected too low results in an increased thickness of the cortex and artifacts in the resulting bone model. On the other hand, if the selected threshold value is too high, it can lead to voids in the bone model (Figure 41.1).23 If translated to a patient‐specific guide, a lower threshold leads to a guide that is too big and might sit loosely on the bone. Alternatively, a higher threshold leads to a smaller guide, which might not fit on the bone because the contact surface of the guide is a negative of the bone model. Manual segmentation allows the user to select or deselect pixels on each slice of the imaging data regardless of their gray value and can be very helpful to correct artifacts after automatic segmentation or to isolate specific bones. After segmentation, the software creates a 3D‐mesh, which can then be exported as a Standard Tesselation Language (STL) file. The resulting file can then be imported into a Computer Aided Design (CAD) software to make further changes or design a patient‐specific guide. The STL file can also be imported into a designated printing software to prepare it for 3D‐printing. Figure 41.1 Different threshold settings and their influence on the resulting 3D model. The same imaging data was segmented with different threshold settings. In (a), the threshold was set too low, resulting in artifacts (arrows). In (b), the threshold was set correctly. In (c), the threshold was set too high, resulting in voids in the resulting bone model. Source: © Paul Schwarzmann. The two most common applications of 3DP‐PSGs are drill guides and saw guides. Drill guides are used to place screws or Kirschner Wires (K‐wires) accurately in the bone for many different applications such as the stabilization of vertebrae,9–18 the treatment of a humeral intracondylar fissure,19 or fixation of an UAP.20 Saw guides are used to make accurate cuts to create specific bone wedges; e.g., in the correction of angular and rotational deformities of the antebrachium4–7 or the femur.8 Many different CAD‐software programs can be used for pre‐procedure planning and guide design. Since there is no clear tendency toward one specific software in the current literature, the decision of which software is used depends on the functions needed for a specific application, the costs of the software, the computing power of the PC, and personal preference. A commercial software program that is frequently used is 3‐Matic (Materialise, Leuven, Belgium).25,32,33 Free software programs that can be used are Meshmixer (Autodesk Inc., San Rafael, CA, USA)5,20 or Tinkercad (Autodesk Inc., San Rafael, CA, USA).27 Regardless of which software is used, the basic principles of designing a 3DP‐PSG are the same. However, there are additional approaches on how to plan a surgery three dimensionally than the method described in the following paragraphs. The principle for both, drill and saw guides, is a “press‐fit” on the bone surface allowing the transfer of a surgical plan accurately to surgery. That means that there is only one possible location and orientation of the guide on the bone. After importing the STL file of the bone model in a CAD software, the first step of designing a guide is planning the surgery. This is done by defining either cuts for a saw guide or holes for a drill guide on the bone model. When designing a drill guide, the first step is to digitally place one or several cylinders in the desired position where a hole should be drilled or a K‐wire should be placed (Figure 41.2a). These cylinders stay in this position and are subtracted from the final guide (Figure 41.2b). The diameter of a cylinder should be selected slightly larger (0.1–0.2 mm) than the drill or the K‐wire to avoid friction between the guide and the instrument and to counteract a slight shrinkage of the guide during the printing process. It is also possible to create a hole big enough to fit a standard drill sleeve.34 In addition to the cylinder representing a drill hole, it can be favorable in many cases to add smaller cylinders representing K‐wires to attach the guide to the bone while drilling. It is important that all cylinders are parallel to each other to allow the guide to be removed in surgery without removing the K‐wires. Figure 41.2 Design of a drill guide for the treatment of an ununited anconeal process (UAP). The cylinders in (a) represent two K‐wires and the drill hole (arrow) for a screw to reattach the anconeal process. The cylinders are then subtracted from the final guide (b). Source: © Paul Schwarzmann. The design of a saw guide is more complex than the design of a drill guide and requires a good understanding of the principles of the performed surgery. When designing a saw guide for the correction of an angular limb deformity (ALD), it is essential to understand the Center of Rotation and Angulation (CORA) principles.35 In cases where a contralateral bone with a physiological conformation is present, this bone can be mirrored and used as a reference.4 To define the cuts for a closing wedge ostectomy, the proximal joint surfaces of the affected and the unaffected, mirrored bone model are superimposed. A rectangular cuboid, which is generated in the software, is placed parallel to the proximal joint surfaces representing the guide for the proximal cut (Figure 41.3a). Additionally, a plane can be placed temporarily on the distal aspect of this cuboid to visualize the cut. For a closing wedge osteotomy, this plane should cut through a closing CORA. The cuboid and the affected bone are then combined and duplicated before the distal joint surfaces of the duplicated bone, and the mirrored, unaffected bone are aligned in all planes (Figure 41.3b). When the cuboid of the original affected bone is now separated and combined with the duplicated bone, this results in two cuboids representing the guides for a closing wedge osteotomy. If not previously done, two planes should be placed on the inner side of those cuboids. The cuboids are moved proximally and distally with the respective planes until the planes intersect at the closing CORA (Figure 41.3c). During this movement, the angle and the rotation of the cuboid must not be changed. If the wedge between those planes is now cut out and the cuboids are superimposed digitally, the ALD should be corrected in all planes (Figure 41.3d,e). However, some translation without altering any angle might be necessary to allow enough contact between the cortices. By simulating the osteotomy digitally, it is possible to assess the result, make corrections to the guide, and print the corrected bone for pre‐bending a plate.
41
3D‐Printing in Orthopedics
Introduction
Production of a 3D‐Printed Guide
Imaging and 3D‐Data Acquisition
Guide Design
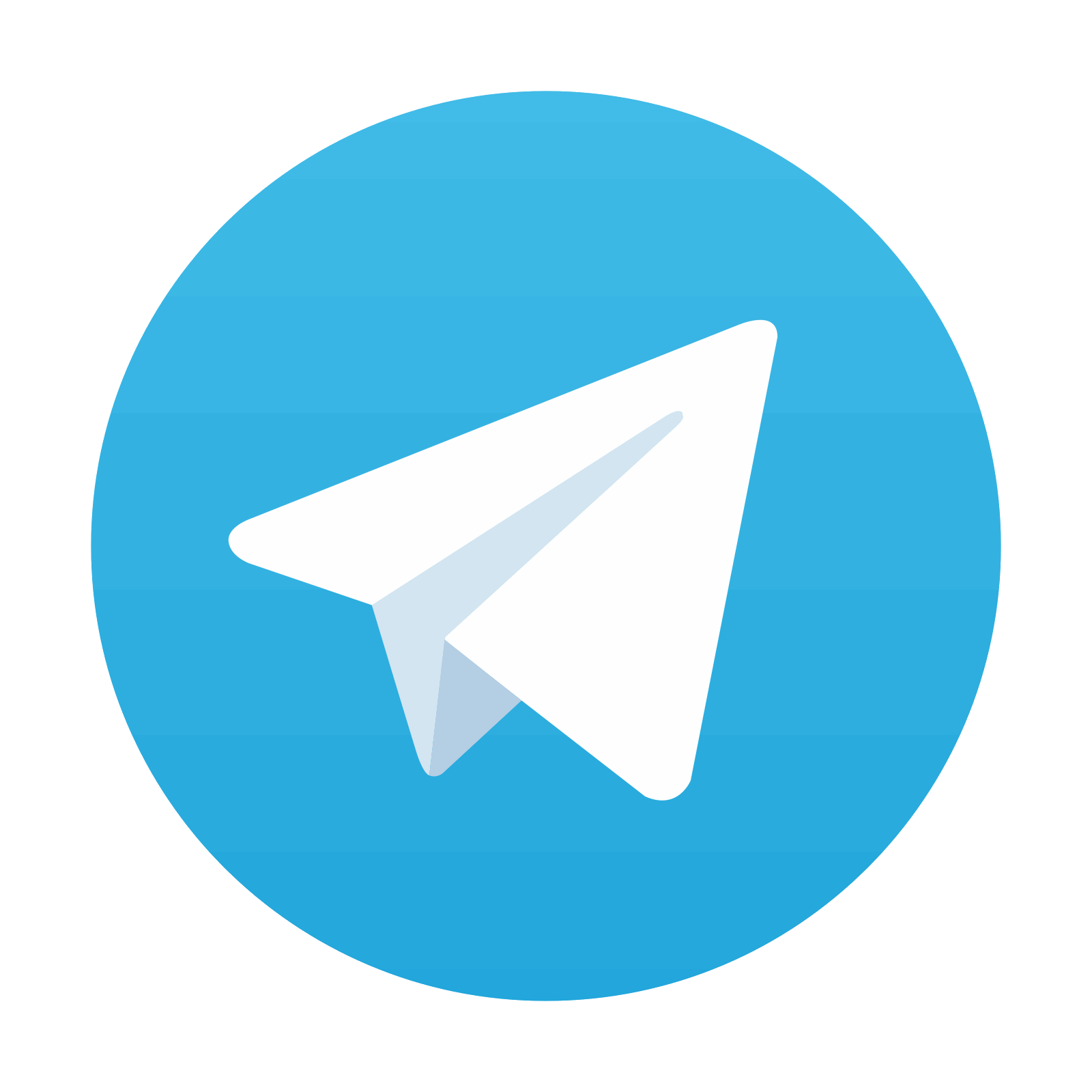
Stay updated, free articles. Join our Telegram channel

Full access? Get Clinical Tree
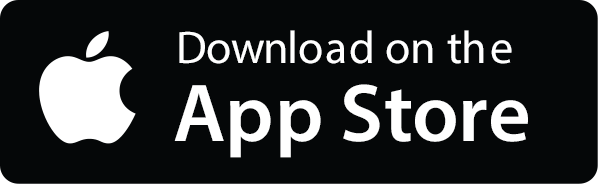
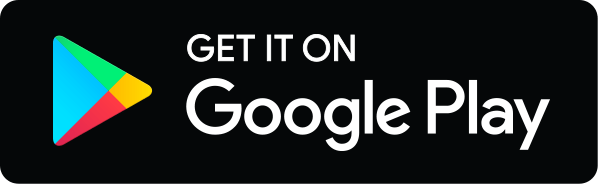