Elodie E. Huguet1, Elizabeth Huyhn2,, and Clifford R. Berry3 1 Department of Small Animal Clinical Sciences, College of Veterinary Medicine, University of Florida, Gainesville, FL, USA 2 VCA West Coast Specialty and Emergency Animal Hospital, Fountain Valley, CA, USA 3 Department of Molecular Biomedical Sciences, College of Veterinary Medicine, North Carolina State University, Raleigh, NC, USA The use of cross‐sectional imaging modalities, such as computed tomography (CT) and magnetic resonance imaging (MRI), has changed the landscape of diagnostic imaging in veterinary medicine over the past several decades. This chapter will provide an overview (not meant to be comprehensive) of the basics in acquisition and interpretation of these cross‐sectional imaging techniques. Computed tomography is a diagnostic imaging modality that creates transverse images of a patient without superimposition of other anatomic structures. The field of view can be selected to give detailed transverse images of the internal organs and areas of interest. The transverse images made during a CT study can also be reconstructed into sagittal, dorsal, and oblique imaging planes and also used to create three‐dimensional images of the anatomy of interest. Computed tomographic exams are usually done in anesthetized or heavily sedated patients to prevent motion artifact, unnecessary repeated examinations and consequently reexposure to ionizing radiation for the patient and possibly personnel. With recent advances in technology, a CT can be done relatively quickly, which is beneficial for a veterinary patient under general anesthesia. CT is often the best imaging modality for detecting a variety of neoplasms since the images allow confirmation of the presence of subtle abnormalities that are potentially not seen on radiographs. CT can also determine size and location for surgical and radiation therapy planning. Iodine‐based contrast media are categorized according to osmolarity (high, low, or iso‐), ion formation ability (ionic or nonionic), and the number of benzene rings within the chemical structure (monomer and dimer) [1]. The most commonly used contrast medium for CT in the United States is nonionic, iodinated contrast medium such as iohexol (Omnipaque®) or iopamidol (Isovue®). Nonionic, iodinated contrast agents cause less discomfort and fewer adverse reactions compared with ionic agents. Adverse reactions to nonionic iodinated contrast medium are rare. When nonionic iodinated contrast medium is given intravenously, adverse reactions include but are not limited to twitching, sinus tachycardia, supraventricular tachycardia, and hyperventilation [2]. When this medium is given intrathecally, adverse reactions include alterations in heart rate and respiratory rate, prolonged apnea, muscle fasciculations, muscle rigidity, seizures, and worsening of neurologic condition [3]. Similar to radiographs, CT also exhibits variable absorption of x‐rays depending on the physical density of the tissues being imaged. The unit used to express CT values is standardized from the physical densities of tissues compared with water and is called the Hounsfield unit (HU). HUs are obtained from a linear transformation of measured attenuation coefficients. An attenuation coefficient is quantified as the measure of how easily a density of material can be penetrated by an x‐ray beam. It quantifies the weakening of the beam when it passes through the material. The linear transformation of the attenuation coefficients is based on the subjectively assigned densities of air and pure water. The radiodensity of distilled water and air at standard temperature and pressure is 0 HU and −1000 HU, respectively. On a CT image, the scale can run from −1000 HU for air to ≥2000 HU for dense osseous structures (Figure 3.1). FIGURE 3.1 Hounsfield units (HU) scale. Note the HU range for different anatomic structures. The CT image is divided into an array of pixels in the x‐y imaging plane. The pixel, short for picture element, is the basic unit of the displayed 2D image. Each pixel represents a voxel, or volume element, which is a 3D volume of tissue described by x, y and z dimensions. Each voxel attenuates the x‐ray beam based on the average density of the tissue contained within it. Based on this attenuation, a gray scale value is assigned to the representative pixel in the image. Window level (WL) is the CT number or HU at the midpoint of the gray‐scale display window. The WL is set at the attenuation of the structure being assessed. For example, if bone is being assessed, the WL must be high (Table 3.1). Window width (WW) determines the contrast of an image, with narrower windows resulting in greater contrast [4]. The WW is selected based on what is being compared. If the attenuation of the structures being compared is widely variable, the WW is wide. If the attenuation of the structures being compared is similar, the WW is narrow (Figure 3.2). TABLE 3.1 Window width and window level in Hounsfield units (HU). FIGURE 3.2 Example of window width (WW) and window level (WL). Note that arbitrarily the WL is set at 100 HU and the WW is 300 HU, there is 150 HU above and 150 HU below the gray scale (150 + 150 = 300). In this case, greater than +250 HU will be hyperattenuating (toward the white part of the image contrast scale) and less than −50 HU will be hypoattenuating (toward the black part of the image contrast scale). Initial CT images are acquired in the transverse or axial plane using a volumetric data set; in other words, anatomy is scanned in the x‐y plane in relation to the bore of the CT machine (z‐axis of the patient). These sets of initial axial images contain information in three dimensions and are used to reconstruct images to be displayed in different planes (Figure 3.3). In veterinary medicine, sagittal and dorsal plane reconstructed images are typically made from the volumetric data set of axial images at the CT computer prior to review of the data set. This data set (raw data) typically consists of axial images with a section thickness of ≤1 mm, preferably with an overlapping interval as the spatial resolution of the sagittal or dorsal plane images is usually reduced compared to the axial plane [5]. The in‐plane pixel dimensions approximate the x‐y‐axis resolution, but the slice thickness limits the z‐axis resolution. Sagittal and dorsal multiplanar reformatted (MPR) images combine the x‐ or y‐axis dimensions of the CT image with image data along the z‐axis, and therefore a mismatch in spatial sampling and resolution occurs during reconstruction with significantly thick slices (3, 5, or 10 mm slice thickness) [4]. FIGURE 3.3 Skull CT of a dog in bone algorithm. A: Transverse plane of the skull at the level of the tympanic bullae. B: Dorsal plane of the skull at the level of the cribriform plate. Note the dotted line demarcating the region where the transverse plane intersects from Figure 3.3a. C: Sagittal plane of the skull along the midline. Note the dotted line demarcating the region where the transverse plane intersects from Figure 3.3a. Multiplanar reformations enable images to be displayed in a different orientation from the original one. These multiplanar reformations include maximum‐intensity projection (MIP) (Figure 3.4), minimum‐intensity projection (MinIP) (Figure 3.5), surface rendering (Figure 3.6), volume rendering (VR) (Figure 3.7), and virtual endoscopy (Figure 3.8). FIGURE 3.4 Maximum‐intensity projection (MIP) of the thorax in a dog in the dorsal plane at 32 mm slice thickness. Note the increased conspicuity of the hyperattenuating tissues of the bone, heart, and pulmonary vasculature after contrast medium administration. FIGURE 3.5 Minimum‐intensity projection (MinP) of the thorax in the same dog as Figure 3.4 in the dorsal plane at 32 mm slice thickness. Note the increased conspicuity of the hypoattenuating tissues of the bronchi and pulmonary parenchyma. FIGURE 3.6 Surface rendering. Hyperattenuating values are selected for this surface rendering with visualization of the osseous structures and organs with contrast medium enhancement. FIGURE 3.7 Volume rendering (VR) image of the thorax of a dog using Horos®, an open source media image software (HorosTM, https://horosproject.org/). Note the visualization of the heart and thoracic vasculature mimicking a lateral thoracic radiograph. FIGURE 3.8 Virtual endoscopy of the carina of a dog using Horos. Note that the top left image is a transverse plane, the top right image is a dorsal plane, and the bottom left image is a sagittal plane, all denoting the pink caliper and green cross‐hairs to delineate the carina as the region of interest. The bottom right is the image produced using the three planes to create a 3D intraluminal image of the carina, mimicking a tracheoscopy/bronchoscopy image. Maximum‐intensity projection enables the evaluation of each voxel (volume element within the image) along a line from the viewer’s eye through the volume of data and to select the maximum voxel value, essentially transforming a two‐dimensional image into a three‐dimensional image with increased conspicuity of the most attenuating structures, such as bone and contrast medium [6]. MIP is helpful when assessing the pulmonary parenchyma for pulmonary metastatic disease. The relative attenuation of a pulmonary metastatic nodule is increased compared to normal aerated pulmonary tissue, thereby increasing its conspicuity on MIP images.
CHAPTER 3
Computed Tomography andMagneticResonanceImaging
Overview
Computed Tomography
Overview: Uses and Advantages
Contrast
Basic Physics
Structures of interest
Window width (HU)
Window level (HU)
Lung
1500
−400
Soft tissue
400
+50
Bone
1600
+500
MPR Reconstructions and 3D Renderings
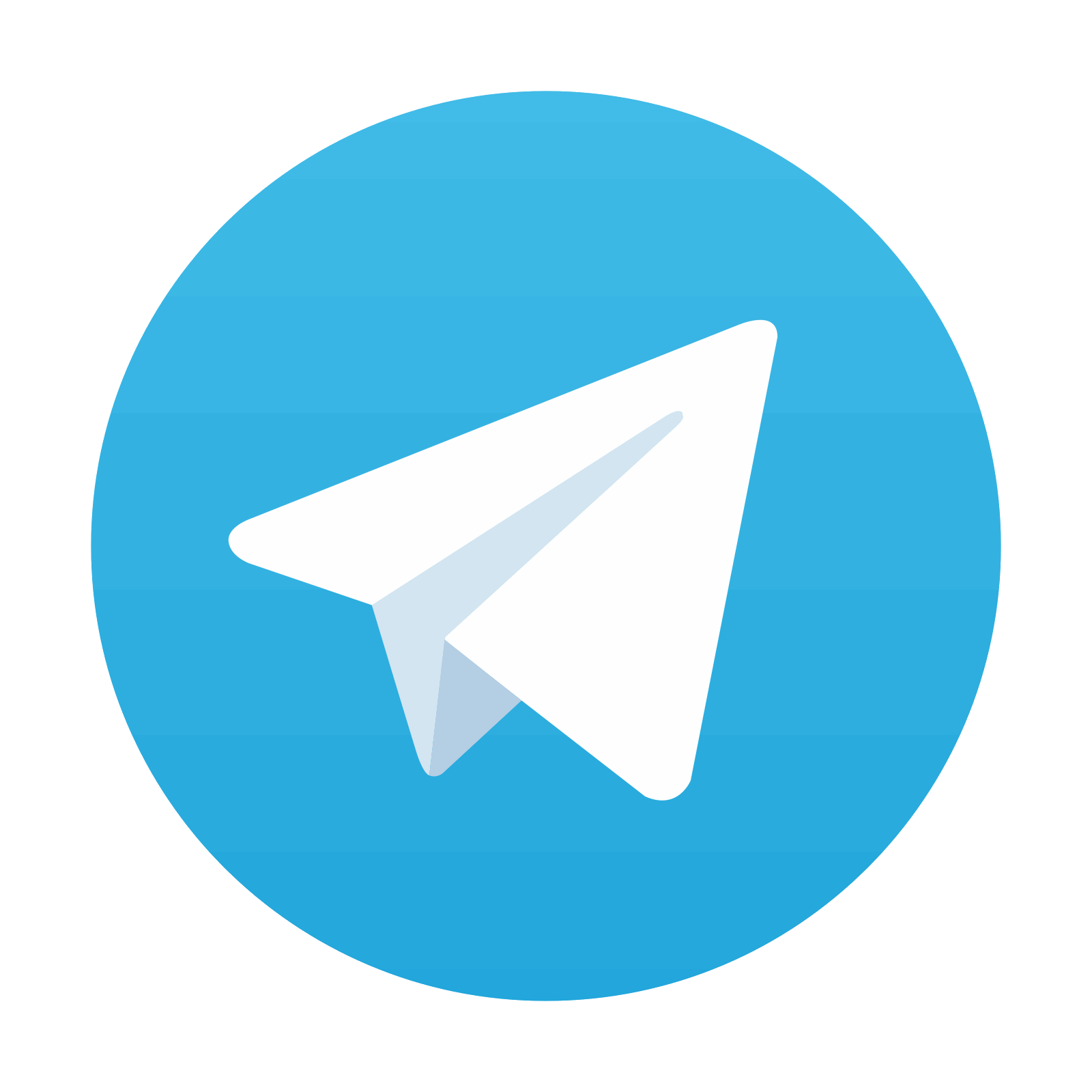
Stay updated, free articles. Join our Telegram channel

Full access? Get Clinical Tree
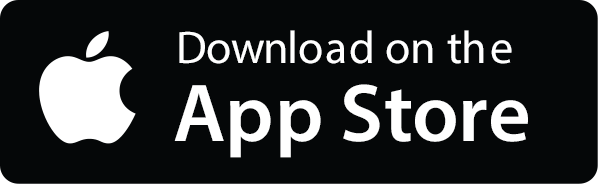
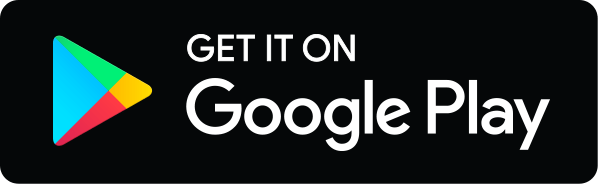