CHAPTER 22 Christine Theoret, DVM, PhD, Diplomate ACVS This chapter focuses on some of the more promising adjunctive and novel therapies developed for or adapted to the horse, based on the specific underlying characteristics that govern wound repair in this species. The choice of topics covered in this chapter is based on the volume and quality of data published in the veterinary medical literature pertaining to each of these adjunctive approaches since the publication of the second edition of this textbook. Problems frequently develop during wound repair in horses and may lead to extensive scarring with adverse effects on cosmesis and on function, often by causing lameness. In addition to welfare concerns, defective healing represents an important economic burden to the equine industry because most horses are destined to an athletic career (the reader is referred to Chapter 1 for more information on the economic consequences of wounds in horses). Consequently, the search for innovative adjunctive approaches to accelerate and improve the quality of repair is paramount. The choice of topics covered in this chapter is based on the volume and quality of data published in the veterinary medical literature pertaining to each of these adjunctive approaches since the publication of the second edition of this textbook. Although similar data are not available for the veterinary industry, the global market for wound care in humans is expected to reach US$18.3 billion by 2019, growing at a compound annual rate of 3.2% from 2014 when it totalled US$15.6 billion.1 A particular niche within this market comprises advanced wound‐care products, which include specialized dressings, bio‐active wound‐care products, such as skin substitutes, as well as therapeutic devices that use negative‐pressure wound therapy or oxygen and hyperbaric oxygen equipment, to name a few. In 2013, the global market for advanced wound care had an estimated value of US$6.2 billion, having grown by 5% since 2012.2 In our quest to improve the success of wound management in horses, extrapolating the results of preclinical studies (in laboratory animals) and clinical trials (in humans) to equine patients is tempting. One must bear in mind, however, that due to the unique nature of wound healing in the horse (reviewed in Chapters 1, 2, and 15), therapies beneficial to other species may not be suitable to equids. Although providing innovations in wound management to the equine practitioner is crucial, many products available to veterinarians rely heavily on anecdotal evidence, and their true effectiveness has yet to be thoroughly evaluated. This chapter focuses on some of the more promising adjunctive and novel therapies developed for or adapted to the horse, based on the specific underlying characteristics that govern wound repair in this species. A wound, when first examined, may be several hours old or perhaps a few weeks old. The different phases of repair should be recalled prior to designing the treatment regimen, keeping in mind that the approach must be dynamic. Indeed, accelerating and/or improving repair are not as simple as applying a single treatment to the wound and maintaining it for the duration of the healing process. A robust yet transitory inflammatory response to injury cleanses the wound in an effort to stave off infection. Moreover, inflammatory cells, along with the platelets that predominate during the coagulation phase, orchestrate the initiation and maintenance of the proliferative phase of repair through the release of cytokines and growth factors. Given that the inflammatory response to wounding is weak but protracted in horses and correlates with the development of chronic, indolent wounds and exuberant granulation tissue (EGT), normalizing the inflammatory response might enhance certain aspects of repair and prevent complications.3 One way to curtail the inflammatory phase is to debride the wound to demarcate non‐viable tissue, particularly when necrosis, exposed cortical bone, or frayed tendons are present because non‐viable tissue facilitates bacterial colonization. Conventional wound debridement involves irrigation and sharp resection. Although low‐pressure irrigation fails to dislodge contaminants effectively, high‐pressure irrigation might damage wounded tissues. Moreover, with sharp debridement, there is a risk of removing viable tissue or leaving necrotic tissue in the wound, especially when the margin between the two is ill defined. An alternative method of wound debridement relies on the use of maggots. Maggots have been used to treat wounds for centuries,4 and nowadays, larval therapy is generally categorized as a “biosurgical” therapy. Maggot debridement therapy (MDT) for human5 and veterinary medical purposes6 uses freshly emerged, germ‐free larvae of the common green bottle fly, Lucilia sericata, produced by specialized centers. At the time of writing, these include Monarch Labs (North America), Polymedics Bio Products (Belgium), BioMonde (UK), Neocura (Germany), Japan Maggot Company (Japan), and the Department of Medical Entomology of the Westmead Hospital (NSW, Australia). The action of maggots on wound healing is attributed to debridement via the production of potent proteolytic enzymes,7 up‐regulation of the expression of genes involved in wound healing,8 an antiseptic effect,9 and an inhibitory effect on the formation of biofilm.10 During the process of dissolving fibrin and necrotic tissue, maggots also destroy and digest bacteria, and the excretions/secretions of L. sericata possess significant antifungal properties.11 In relation to the rising incidence of antibiotic resistance, the action of maggots against methicillin‐resistant Staphylococcus aureus (MRSA) is of particular interest.12 Sterile maggots can be applied to a wound using a direct (free‐range) or indirect (contained) contact method. With the direct contact method, maggots are applied on to the wound with a dressing fixed to the surrounding healthy tissue (Figure 22.1). After placing the maggots on to the wound, a nylon mesh is fixed to the dressing to confine the maggots within the wound and to prevent them from escaping. Using the indirect contact method, maggots are supplied within a closed polyester net filled with absorbent hydrophilic polyurethane foam (Figure 22.2).13 Although the indirect contact method has obvious practical and esthetic advantages over free‐ranging maggots, a study of 64 human patients with signs of gangrenous or necrotic tissue showed that a better outcome is achieved with the direct contact method of application than with the indirect contact method.14 The authors of an equine study using MDT15 reached a similar conclusion and considered the direct contact method superior. An additional benefit of free‐range maggots is the reduced cost of therapy. Figure 22.1 Maggots used in the direct contact manner (free‐range maggots). (a) Larvae being prepared for application in the direct contact manner. (b) Larvae are applied directly to a penetrating injury of the foot. Courtesy of Dr. O.M. Lepage. Figure 22.2 Maggots and pieces of foam within a closed polyester net to be used in the indirect contact (contained) method. Pieces of foam offer a physical environment to stimulate the activity and development of the maggots whilst assisting with management of exudate. Courtesy of Dr. O.M. Lepage. In horses, MDT is described as an alternative approach to the management of septic navicular bursitis, laminar necrosis associated with complicated laminitis,16 and subsolar infection‐associated osteomyelitis of the distal phalanx.4 Maggot debridement therapy has also contributed towards a successful outcome in limb lacerations, including those leading to chronic non‐healing wounds, in orthopedic infection free of bony sequestrae, in various soft‐tissue abscesses, in supraspinous bursitis, and in dehiscence of the linea alba following exploratory celiotomy.15 In a retrospective study of 41 equids treated with MDT, 38 had a favorable outcome less than 1 week after initiation of therapy. Lesions enrolled in this study were subacute or chronic and had been managed in diverse ways prior to receiving MDT. When considering MDT, the practitioner should keep in mind that free drainage of exudate is required and that the maggots must have an adequate supply of oxygen. A preliminary surgical debridement usually precedes MDT, though this may not be necessary in the absence of foreign bodies and/or organic matter in the wound. In some cases, after 3–4 days of MDT, a second treatment is required to reach the desired degree of debridement, disinfection, and fibroplasia (i.e. healthy granulation tissue filling the wound). Orthopedic shoeing, stent bandaging, and non‐occlusive sleeve bandaging, typical to MDT, are adapted for each type of wound and anatomic location6,12 (Figure 22.3, Figure 22.4). Because maggots, after being applied to a wound, contain viable bacteria and continue excreting these, they are regarded as medical waste and must be disposed of after use according to local regulations. Figure 22.3 A chronic abscess has developed over the tuber coxae subsequent to a fracture leading to bony sequestration. (a) Maggots are placed in the cavity created by surgical debridement and removal of the bone sequestrum. (b) A stent bandage made from a baby diaper is sutured over a non‐occlusive wound dressing. Courtesy of Dr. O.M. Lepage. Figure 22.4 Use of MDT and application of a non‐occlusive “sleeve bandage” in a horse with a wound on the distal aspect of the limb. (a) The net provided by the manufacturer is placed in a 360° bracelet and attached to the limb, distally, with an elastic adhesive bandage, before pouring on the maggots. (b) The net is then fixed to the limb, proximally, with an elastic adhesive bandage, to seal the treatment area. (c) A cotton pad is applied on top to absorb secretions, and a rigid prosthesis is added, if necessary. Courtesy of Dr. O.M. Lepage. In conclusion, based on clinical observations in equine medicine and a systematic review of MDT for chronically infected wounds and ulcers in humans,17 MDT is recommended for use in horses to ensure debridement and disinfection and to enhance healing of subacute and chronic, non‐healing wounds, when complete surgical debridement is difficult or impossible, and to enhance healing of obviously infected wounds. Although a robust inflammatory response is required for prompt initiation of the subsequent proliferative phase of healing, it is the products of the inflammatory cells, rather than the cells themselves, that mediate the desired effects. It is, therefore, tempting to be drawn to the promise of bioactive molecules, such as cytokines/growth factors released by inflammatory cells and/or platelets, as therapeutic tools. Complex combinations of cytokines involved in wound healing variably stimulate migration, attachment, proliferation, and differentiation of endothelial cells, epithelial cells, and fibroblasts (the reader is referred to Chapter 1 for more information on cytokines). In spite of a drive, in the past two decades, to ascertain the therapeutic effects of purified forms of specific cytokines in the clinical management of non‐healing wounds in humans, the overall conclusion of a recent critical analysis of the literature is that larger randomized controlled trials are needed to document side‐effects and to confirm efficacy and long‐term outcomes.18 The intricate mechanism of action of cytokines has yet to be fully elucidated. Because these molecules network both spatially and temporally amongst themselves and also with cells and the surrounding matrix, addition of an isolated cytokine might disturb the fine balance. Furthermore, the exogenous cytokine may not exert its anticipated impact because interaction with endogenous counterparts (e.g., degradative enzymes) could abrogate its in vivo effect. As such, expecting an individual molecule to enhance repair is illusory, particularly if it is not known whether a deficiency of this specific molecule is at the root of the problem. Instead, as is the case with dressings that must vary to suit the needs of each phase of repair, a dynamic “combination” therapy based on the use of a physiologically natural mixture of cytokines may be most appropriate. With the aim to provide combination therapy, investigators have turned to “platelet concentrates,” the preparation of which may be achieved using various methods, each leading to a specific product that differs from the others in its biologic effect and potential use. In an effort to dispel confusion and better discriminate between the therapeutic successes and failures reported in the literature, a classification scheme was recently proposed wherein platelet concentrates are grouped in four main categories: pure platelet‐rich plasma (P‐PRP), leukocyte‐rich PRP (L‐PRP), pure platelet‐rich fibrin (P‐PRF), and leukocyte‐rich PRF (L‐PRF).19 In the latter two categories, activation of platelets and polymerization of fibrin are triggered artificially to generate a high‐density fibrin clot that can serve as a biologic healing matrix by supporting cellular migration and release of cytokines. The true impact of leukocytes in platelet concentrates is unknown and remains the subject of controversy. Some authors recommend eliminating leukocytes, whereas others emphasize the need for leukocytes in platelet concentrates because of their anti‐infectious and immunoregulatory activities, critical to repair.19 The concern that L‐PRP or L‐PRF might induce negative effects (via activation of inflammation) does not apply to the management of acute open wounds of horses where stimulation of a robust inflammatory response is, in fact, desirable.3 Normal horse blood contains approximately 142 000 platelets/μL, a far weaker concentration than that found in other mammals, including humans.20 The active secretion of proteins by platelet α‐granules begins within 10 minutes of clotting, with more than 95% of the presynthesized cytokines secreted during the first hour. After this initial burst, platelets synthesize and secrete additional proteins for the balance of their life (5–10 days).21 The addition of thrombin and/or calcium chloride (CaCl2) simulates natural clotting and, therefore, causes the release of several cytokines, in particular transforming growth factor beta (TGF‐β), platelet‐derived growth factor (PDGF), and vascular endothelial growth factor (VEGF), as well as microbicidal molecules beneficial to wound healing. Thus, the principal therapeutic advantage of platelet concentrate over isolated growth factors is that it represents a natural mixture of stimulatory and inhibitory mediators designed to have synergistic biologic effects within the environment of a wound. Indeed, recent in vitro studies have shown that PRP promotes proliferation of human dermal fibroblasts and causes differentiation of the fibroblast into contractile myofibroblasts, thereby promoting wound contraction.22 Moreover, activated equine platelets, such as those found in PRF, are capable of releasing reactive oxygen species that can assist in killing bacteria,23 including methicillin‐resistant Staphylococcus aureus.24 The optimal content of platelets and leukocytes required to achieve a desired biologic effect in horses has not been determined and no doubt varies according to the intended therapeutic purpose. Change in fold of platelet numbers, compared to numbers in whole blood, and absolute platelet numbers are used to estimate adequacy of platelet concentration, with the latter being the best approximation of the concentration of cytokines expected within a tissue after platelet degranulation.25 Platelet concentrations in human PRP commonly range from a 3‐ to 5‐fold increase over whole blood26 or a minimum concentration of 300 000 to 1 000 000 platelets/μL. Extrapolating appropriate concentrations from the human literature may be inappropriate because of differences in average platelet counts and platelet physiology between species, and, consequently, the most appropriate concentration of platelets in equine PRP has yet to be established. Thus, the current “clinically acceptable” value of 250 000 platelets/μL25 is merely a suggestion. A study has shown that at least four different methods of counting platelets are suitable for quantifying platelets in equine PRP, including optical scatter, impedance, hand counting, and fluorescent antibody flow cytometry.27 When anticoagulated blood is centrifuged, three layers become evident: the bottom layer, comprised of red blood cells; the middle layer, comprised of platelets and white blood cells (the buffy coat); and the top, plasma layer. Numerous systems to produce autologous platelet concentrates, originally developed for human patients, are commercially available but often expensive.29 Moreover, given the substantial interspecies differences in platelets, clinicians should not rely on the manufacturers’ data relating to human patients to select the most appropriate method for horses.30 In an effort to improve accessibility to this therapy for all horse owners, inexpensive methods of tube centrifugation have been developed and tested; these appear sufficient to generate an autologous product, free from bacterial contamination, when conducted in a clean environment.31 Dahlgren’s team found that the use of a sterile, skirted, polypropylene, 50‐mL conical centrifuge tube, spun for 15 minutes at 720 Xg, concentrates the platelets by more than 2.5‐fold.25 The yield of PRP using a method of tube centrifugation is approximately 10% of the volume of whole blood drawn. After the PRP is prepared it is stable, in the anticoagulated state, for 8 hours or longer. It was recently shown that, regardless of the stimulus, release of PDGF and TGF‐β is maximal by 0.5–1 hour after clotting and very little release is observed after 24 hours.32 The most effective and inexpensive method of activating PRP for use in horses seems to be the addition of 23 mM of CaCl2.33 Using autologous thrombin is not recommended in horses because it is less effective than CaCl2 in eliciting release of cytokines from the platelets. That said, intrinsic factors, such as the breed, gender, and age of the horse, may affect the cellular content and release of cytokines from concentrates of equine platelets.34 Platelet concentrate can be delivered to the wound with a commercial spray applicator or with a dual‐injection system (one syringe contains the PRP and the other contains the clotting agent), or it can be activated directly in the centrifuge tube after which the resulting gel (PRF) is applied to the wound. A recent review of controlled studies (nine randomized, six prospective, and two retrospective) assessing the clinical outcome of acute wounds in humans treated with platelet‐rich plasma found that PRP accelerates healing of acute wounds.36 A handful of studies have measured the therapeutic effect of platelet concentrate on experimental wounds in horses. Platelet‐rich plasma gel accelerated epithelial differentiation and enhanced collagen organization in wounds on the neck healing by primary intention,37 and accelerated repair, induced fibrosis, and may have provided antibacterial activity in deep, second‐degree burns on the rump.38 In our experience, PRP gel applied topically to acute, full‐thickness skin wounds on the distal aspect of the limb favors fibroplasia, and therefore, we recommend applying PRP gel to acute wounds in which a large deficit must be filled with new granulation tissue.35 Moreover, based on our experience, we conjecture that application of PRP to chronic wounds, after the wound has been thoroughly debrided, might also be beneficial. In conclusion, scientific data supporting the use of autologous platelet concentrate in horses to assist repair and regeneration of injured tissues is promising. That said, one must keep in mind that platelet concentrates are biologic products, rather than drugs, and therefore, their uniformity cannot be guarenteed, especially given the wide range of methods whereby they are prepared. In an effort to ensure maximum therapeutic efficacy, clinicians should verify the quality of the product by measuring its platelet concentration after it has been prepared and prior to using it on a wound. Because this technology necessitates minimal investment or expertise, it should be considered as part of an equine practitioner’s armamentarium for wound management. Another approach shown to positively modulate the inflammatory response to wounding involves the therapeutic use of honey. Wild honey combined with animal fats and lint was used to promote second‐intention wound healing as far back in time as ancient Egypt.39 Since then, in many parts of the world, different varietals of honey have been used to enhance wound healing. The appeal of honey as a therapeutic agent faded after the introduction of modern antimicrobial agents. More recently, the appearance of antibiotic resistance has revived interest in the antimicrobial properties of honey. Although most research has focused specifically on the antibacterial activity of different varietals of honey, honey appears to have a wider therapeutic scope in wound management.40,41 Honey has a broad range of bioactivities, including antimicrobial activity, osmotic and antioxidant activity, and an ability to modulate the inflammatory response to wounding. These combined bioactivities result in enhanced autolytic wound debridement, a deodorizing action, and an immunomodulation, that together reduce pain, edema, and exudate. Ultimately, the effect is to promote wound healing and minimize scarring.40 Despite the reported range of bioactivities of honey, a recent review of clinical trials in humans showed limited evidence of any consistent effects across different types of wounds.42 Dismissing the value of honey as a wound healing agent is premature, however, because new bioactive components of honey are still being discovered.43–45 The diverse spectrum and concentrations of these bioactive components within different varietals of honey means all honeys do not behave equally.43–45 Whether or not different varietals of honey have variable effects when applied to dissimilar wounds during the various phases of healing has yet to be elucidated. To date, research has focused predominantly on the antimicrobial properties of honey used for wound management in humans. Honey has a broad spectrum of activity against bacteria and fungi, but emerging evidence highlights a remarkable variability in antimicrobial potency between varietals of honey.40,46 A high sugar content and low pH are common to all honeys. Many bacteria cannot thrive in an acidic environment, and the low pH of honey also reduces the activity of bacterial proteases within the wound,47 thereby protecting cytokines and growth factors important to healing. The high sugar content of honey creates an osmotic gradient causing fluid shifts that: (1) shrink the bacterial cell wall and inhibit growth; and (2) provide a moist environment and nutrients required by healing tissues as fluids inflow from adjacent tissues and the circulation.40 Glucose oxidase, produced by bees, is present in most honeys. Its antibacterial activity, via the production of hydrogen peroxide,48,49 increases exponentially when it is diluted with wound exudate.48,50 Damaged cells and some bacteria can produce substantial amounts of catalase that destroys hydrogen peroxide, thereby contributing to the variable antibacterial activity of honey in different types of wounds.51 Encouragingly, there are currently no clinical reports of acquired antimicrobial resistance to honey; this is probably due to the multiple synergistic activities that give honey its antimicrobial properties.45 Nevertheless, a recent in vitro study demonstrated that suboptimal concentrations of Manuka honey can allow resistant bacterial wound pathogens to emerge,43 highlighting the need to maintain adequate concentrations of an efficacious honey at the wound–dressing interface. Honey derived from the Leptospermum species of plants has been found to have superior antibacterial activity compared to other honeys, even in the presence of inhibitory concentrations of catalase.51,52 The Leptospermum species include approximately 80 plants growing primarily in Australia and New Zealand. Honey from the Manuka bush (L. scoparium) found in New Zealand is the most extensively studied, and its antibacterial activity has been standardized for commercial production. Hydrogen peroxide is not responsible for the antibacterial activity of Manuka‐derived honey. Rather, this honey’s antibacterial activity is attributed to methylglyoxal (MGO), derived from dihydroacetone in the nectar of the Manuka flower and referred to as the Unique Manuka Factor (UMF), displayed on commercial Manuka honey products.53,54 The UMF is an antibacterial rating, determined by comparing the antibacterial activity of a honey to a standard concentration of phenol: the higher the UMF rating, the greater the spectrum and efficacy of the antibacterial properties of the honey. Generally speaking, honey with a UMF rating greater than 10 is associated with better therapeutic outcomes,55–57 but as the UMF rating increases, so does the cost of treatment. Although biofilms appear to interfere with the antibacterial activity of many honeys, the MGO in Manuka honey is effective in preventing formation of biofilms and disrupting those that are established,58–60 although higher concentrations are required to achieve the latter.61 Veterinary protocols of wound management that incorporate the use of honey describe using products that range from inexpensive honeys intended for human consumption to standardized, medical‐grade, sterilized Manuka honey (Figure 22.5). Carnwath et al. recently measured the antimicrobial activity of various varietals of honey against 10 common equine wound bacterial isolates, including methicillin‐resistant Staphylococcus aureus and Pseudomonas aeruginosa.45 Of 29 tested products, over 60% were found to be contaminated with aerobic bacteria or fungi, emphasizing the importance of using only sterilized honey for wound treatment. Sterilization can be achieved with gamma‐irradiation, which has no significant effect on the antibacterial properties of honey, as opposed to heat treatment, which does.62 Eight products were effective against all 10 bacterial isolates at concentrations varying from <2% to 16% (v/v); these products included Manuka honey (UMF rating greater than 10), commercial wound products and dressings incorporating medical‐grade Manuka honey, and some commercially available monoculture honey from local suppliers.45 Figure 22.5 Manuka honey‐impregnated tulle dressing from Advancis Medical. Courtesy of Dr. D. Knottenbelt. Other areas of research have focused on the healing properties of honey, in particular its ability to modulate the inflammatory response to ultimately promote repair.63 Some varietals of honey were shown to stimulate inflammation of acute wounds, thereby up‐regulating the production of cytokines governing proliferation of fibroblasts and endothelial cells necessary for fibroplasia and angiogenesis.64 For example, a component of Manuka honey was shown to stimulate the release of tumor necrosis factor (TNF)‐α, interleukin (IL)‐1β, and IL‐6.65,66 Conversely, other varietals of honey may inhibit the same cytokines, thereby diminishing the inflammatory response in microenvironments where inflammation is uncontrolled, as is the case in chronic wounds.63,67 The immunomodulatory activity of different varietals of honey is complex and requires more study if honey is to be used strategically for the treatment of diverse types of wounds.63 Moreover, the effects of gamma‐irradiation on the healing properties of honey are unknown. Three studies were recently conducted to investigate the effects of UMF 20 Manuka honey on second‐intention healing of experimental, non‐contaminated and contaminated wounds on the distal aspect of limbs of horses.56,68,69 A 100% UMF 20 Manuka honey and a 66% UMF 20 Manuka honey/34% water‐based gel were shown to equally decrease wound retraction when compared to no treatment; wounds remained significantly smaller than untreated wounds up to day 35 of healing (Figure 22.6).56,68 Manuka honey gel decreased inflammation and increased angiogenesis, organization of collagen, and epithelialization in contaminated wounds, as determined histologically.69 The investigators concluded that contaminated wounds on the distal aspect of the limb treated with 66% Manuka honey gel developed a more organized and mature bed of granulation tissue earlier than untreated wounds, although the mechanism underlying this acceleration was unclear.69 When applied daily throughout healing, 66% Manuka honey gel decreased the overall time required for healing by approximately 10%.68 Surprisingly, while experimental contamination of wounds produced the expected transient increase in aerobic and anaerobic bacterial counts, these counts were unaltered by the topical application of Manuka honey.69 The degree of tissue damage and wound contamination achieved using an experimental model is likely not representative of many naturally occurring wounds on the distal aspect of the limb, causing the antibacterial and immunomodulatory effects of honey to be underestimated.69 Figure 22.6 Surgically created wounds on the left and right metacarpi of a horse, 5 days after wound creation. Both wounds were bandaged and bandages were changed daily. (a) This wound was treated daily with Manuka honey. It is filled with healthy granulation tissue and is flush with the skin. (b) This wound received no treatment other than the bandage and shows irregular granulation tissue with areas of persistent fibrin deposits. The wound treated with honey appears to have a more mature and healthy granulation bed compared to the untreated wound. Courtesy of Dr. A. Dart. Figure 22.7 This donkey was presented with a deep bite wound to the nostril/lip area. The wound was initially treated by sharp surgical debridement then irrigated. (a) In view of massive contamination and the presence of residual necrotic tissue, the wound was subsequently treated with locally sourced honey to encourage continued autolytic debridement. (b) Same wound, 22 days later. Courtesy of Dr. D. Frappier. Since the middle of the 20th century, our greatest asset in the management of traumatic wounds and burns has been an improved understanding of the cellular and subcellular events of wound healing, including recognition of the highly interactive role of the extracellular matrix (ECM). Newly developed adjunctive therapies aimed at enhancing biologic processes (e.g., angiogenesis, fibroplasia) and reducing negative events (e.g., edema, infection) can now be used successfully in a variety of ways through pharmacologic, biologic, and mechanical interventions. One of the most commonly used adjuncts in human wound care today is negative‐pressure wound therapy (NPWT); also termed “vacuum‐assisted closure,” “topical negative‐pressure therapy,” and “sub‐atmospheric pressure dressings.”70,71 In the few decades since the original publications on NPWT, the penetrance of this modality in human medicine has been astonishing. Although originally designed (and still used) for chronic wounds, this treatment modality has become the first line of treatment for acute traumatic wounds, including degloving injuries, shear trauma, high‐energy soft‐tissue wounds, and open fractures, as well as for dehisced surgical wounds, skin flaps, free grafts, and abdominal sepsis.72–74 The modality involves sealing the wound from the environment and delivering a controlled sub‐atmospheric pressure to the entire wound. This is accomplished by trimming sterile, open‐cell polyurethane ether foam to the dimensions of the wound, placing it directly on to the wound bed, and covering both wound and foam with an occlusive, adhesive drape. The drape establishes a closed‐wound environment by adhering to the skin surrounding the wound, the hair of which has been closely clipped. A small hole is then cut through the adhesive drape over the foam and connected to a programmable vacuum pump via a fenestrated suction pad and evacuation tubing (Figure 22.8). Once activated, the pump provides continuous or intermittent suction, typically not exceeding –125 mmHg. Fluid produced by the wound is collected into a disposable reservoir canister attached to the pump. The open cell nature of the foam and the cell size (400–600 microns) ensure even distribution of negative pressure to the entire surface of the wound. Figure 22.8 An extensive wound on the medial surface of the thigh and crus of a dog has been dressed with proprietary open cell foam and sealed from the environment by the placement of an adhesive drape. Vacuum is achieved through the fenestrated suction pad that is connected to the vacuum pump by tubing. There is a replaceable canister for collection of wound fluid at the back of the pump (not visible in photograph). Courtesy of Dr. B. Stanley. Early studies on animal models showed that NPWT stimulates the rapid formation of granulation tissue, reduces the size of the wound, and effectively removes fluid from the wound.75,76 NPWT is currently used in all 6000 primary care hospitals in the USA, and is applied to over 90% of wounds of the extremity, encountered in the military arena.77 The widespread use of NPWT is largely due to its huge clinical impact because not all mechanisms of action have been fully elucidated.71 The early appearance of granulation tissue is remarkably consistent with the use of NPWT and concurs with observed increases in fibroblast activity and angiogenesis.75,78 Other identified and validated mechanisms of NPWT include the removal of interstitial fluid (thus reducing wound edema), altered blood flow to the wound and up‐regulated gene expression of cytokines associated with wound healing.70,79–81 Using finite element analysis, the extracellular and cellular components of the wound treated with NPWT have been shown to be subjected to the same degree of micromechanical strain as that which promotes cellular (fibroblast) proliferation in vitro.82 Nevertheless, certain claims of mechanisms of action, such as increased bacterial clearance, remain to be clarified.70,83 More recent developments with this modality include the introduction of different types of foam, including a silver‐impregnated polyurethane foam, and units that allow controlled delivery of topical solutions by alternating instillation and soak periods with the negative‐pressure therapy (referred to as NPWTi).84 These dressings and units are designed to be used to treat severely contaminated wounds, and thus may be of interest to the equine practitioner. A recent study conducted on an equine perfused ex vivo wound model determined that a polyvinyl alcohol foam dressing should be used as the primary dressing when performing NPWT for treating contaminated wounds healing by second intention in horses.85 Similar positive outcomes using NPWT have been documented in veterinary medicine, although the literature is far less extensive. A small number of case reports describe the use of NPWT in single animals of several species, including the horse; the authors of these reports concluded, based on subjective assessment, that NPWT facilitated second‐intention healing and substantially shortened the time to healing. Several case series and reviews on the use of NPWT in various domestic animals and some experimental studies examining the use of NPWT in dogs, have provided a higher level of evidence of the effectiveness of NPWT used to treat wounded animals.86–93 Most recently, a retrospective multicenter study of 50 dogs undergoing open wound management established that time to healing was halved in patients treated with NPWT compared to those treated with a highly absorbent foam dressing (Allevyn Cavity, Smith & Nephew), which in turn healed faster than patients treated with conventional non‐adherent gauze dressing.94 It is clear from these studies that not only is the use of NPWT feasible in veterinary medicine, but, as in wounds of human patients, it also leads to the early appearance of superior‐quality granulation tissue and thus shortens the time to reconstruction (Figure 22.9). Indications for the use of NPWT in veterinary medicine include traumatic wounds (avulsions, degloving, shear injuries), full‐thickness burns, dehiscence of surgical incisions, myofascial compartment syndrome, and chronic non‐healing wounds.86,90,92 NPWT is also excellent for enhancing acceptance of free grafts,87 and it can be used effectively over sutured incisions to splint closures under tension, prevent formation of a seroma, particularly in closed incisional wounds with underlying dead space,95 and minimize edema.92 Negative‐pressure wound therapy is used in all veterinary schools and many specialist facilities in North America.
Innovative Adjunctive Approaches to Wound Management
With contributions from: Olivier Lepage – Maggot debridement therapy; Andrew Dart and Andrea Bischofberger – Honey;Bryden Stanley – Negative-pressure wound therapy; and Judith Koenig – Extra-corporeal shockwave therapy
Summary
Introduction
Innovative adjunctive approaches
Maggot debridement therapy (MDT)
Platelet concentrates
Honey
Negative‐pressure wound therapy
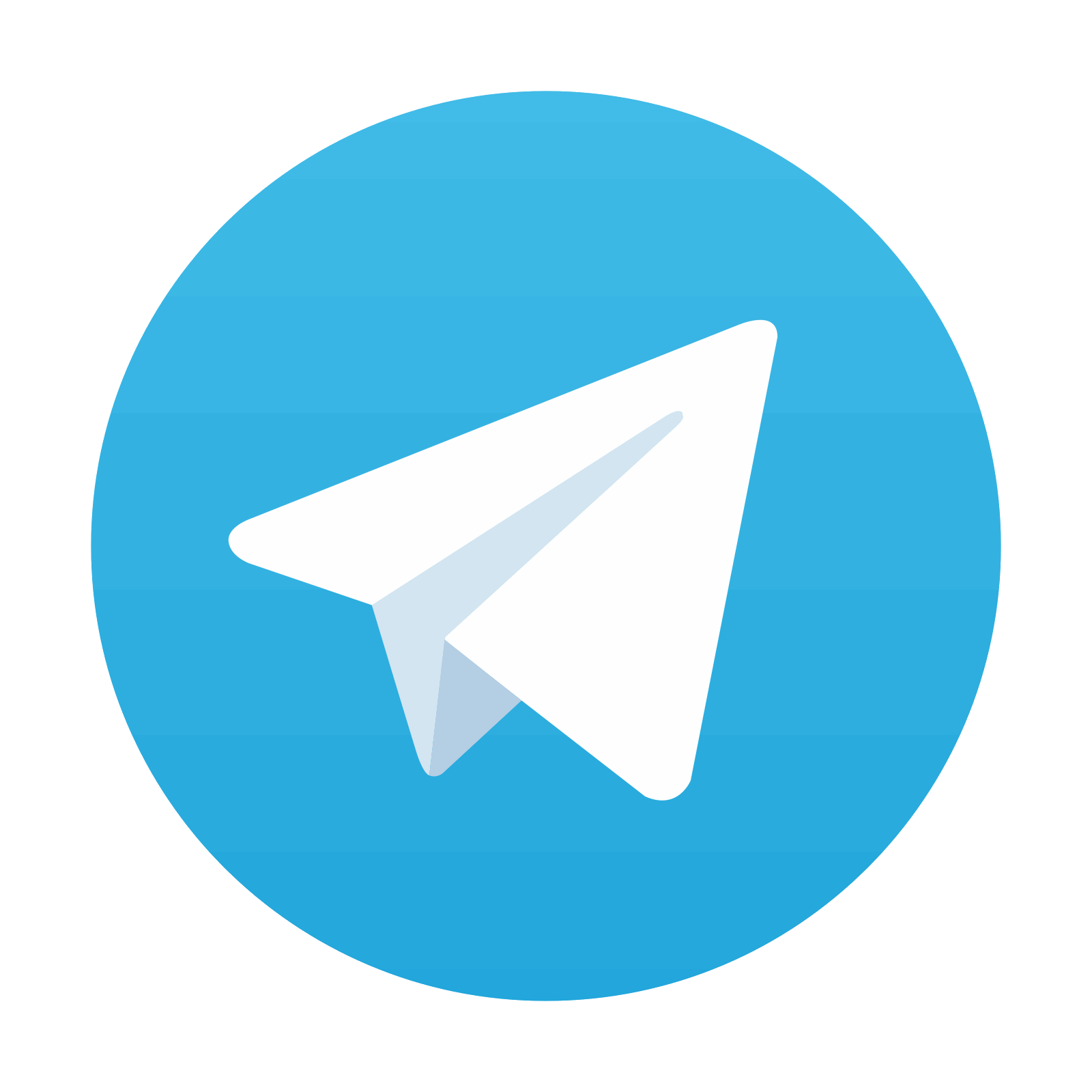
Stay updated, free articles. Join our Telegram channel

Full access? Get Clinical Tree
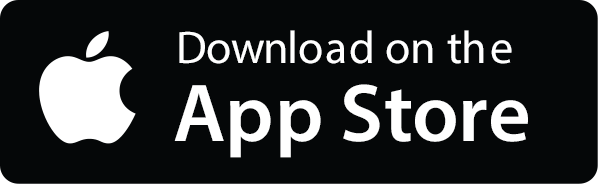
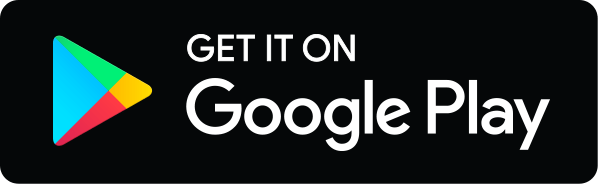