CHAPTER 19 Armi Pigott Lakeshore Veterinary Specialists, Milwaukee, Wisconsin Pain has been defined as an unpleasant sensory and emotional experience associated with actual or potential tissue damage [1]. This unpleasant feeling is conveyed to the brain by sensory neurons, giving information on the location, intensity, and, potentially, the nature of the pain. A variety of systemic alterations occur as a consequence of pain and, when left unchecked, can increase morbidity and mortality in the small animal ICU patient [1–4]. Neurohormonal changes occur in response to pain, with increased sympathetic tone, increased myocardial work and oxygen consumption, increased skeletal muscle flow, and decreased blood flow to the gastrointestinal (GI) and urinary tracts. Circulating adrenocorticotropic hormone (ACTH), cortisol, and norepinephrine are increased while circulating insulin is decreased. These changes contribute to a catabolic state that may decrease or prolong healing. Many painful patients are reluctant or unable to eat or move, contributing further to patient morbidity. There are five neurophysiological phases involved in the sensation of pain: transduction, transmission, modulation, projection, and perception (Figure 19.1). An understanding of these steps is important since certain analgesic and anesthetic drugs can be administered to target a specific step (Box 19.1). Figure 19.1 Schematic of neurological pathways for pain perception. Inflammatory mediators (such as histamine, tumor necrosis factor, interleukins, hydrogen ions) bind to one or more of the specific receptors on the nociceptor. The pain perception impulse is transmitted to the spinal cord segment through the primary afferent nerve fibers of the peripheral nerve (burgundy line) and synapses with spinal cord neurons in the dorsal horn of the spinal cord. Projection neurons (blue lines) from the dorsal horn transmit information to the somatosensory cortex through connections in the thalamus. This relays information regarding the intensity and location of the pain. Other areas of the brain are stimulated and provide descending information that regulates output from the spinal cord and the reaction to pain. In the dorsal horn of the spinal cord (box), the nociceptor afferent (burgundy solid line) releases a variety of neurotransmitters (such as ATP, glutamate, CGRP, substance P) that interact with specific receptors on the spinal pain tract neurons. This facilitates the transmission of pain messages to the brain. The tonically active inhibitory neuron (brown dashed arrow) receives impulses from the brain that act to inhibit the stimulation of the spinal tract pain nerves to slow or terminate the pain cycle through the release of inhibitory neurotransmitters (such as glycine, GABA). The activity of this inhibitory neuron is lessened or lost (disinhibition) during central sensitization. The transduction (phase 1) of a chemical, mechanical or thermal noxious stimulus is mediated by the pain receptors at the peripheral ends of the pain fibers, called nociceptors. These receptors are located in skin, mucosa, deep fascia, and connective tissue of visceral organs, ligaments, muscles, tendons, joint capsules, periosteum, and arterial vessel walls [3,5,6]. Their function is to detect and transmit the location, quality, and duration of a stimulus [3,5–7]. There are many types of nociceptors, each detecting a specific stimulus (such as heat, stretch, vibration, pressure). In the viscera, nociceptors are more sensitive to distension, twisting, and ischemic injury as noxious stimuli. The minimal stimulus required for a nociceptor to generate an electrical signal (an action potential) is called the nociceptor threshold [8]. A variety of chemical substances are responsible for the transduction of a pain signal and can include globulin and protein kinases, arachidonic acid, histamine, nerve growth factor, substance P, calcitonin gene‐related peptide, potassium, serotonin, lactic acid, vasopressin, glucocorticoids, oxytocin, catecholamines, angiotensin, and endorphins/enkephalins [9,10]. The degree and duration of the stimulus determine the number of action potentials per unit of time and the duration of production of these action potentials. Once the stimulus has been converted to an action potential, transmission of the signal occurs (phase 2); the electrical signal is conducted up the afferent sensory nerve fibers to the spinal cord, and depolarizes the presynaptic terminal. The presynaptic terminal interfaces with a network of interneurons and second‐order neurons in the dorsal horn of the spinal cord. Interneurons can either facilitate or inhibit transmission to second‐order neurons. There are two main types of nociceptor fibers which transmit action potentials to the spinal cord. A‐delta fibers (Að) are moderate‐speed (20 m/sec), thinly myelinated fibers which are associated with initial, sharp or pricking, well‐localized pain. C fibers are slower (2 m/sec), thin, unmyelinated fibers which are associated with a dull, aching, throbbing, diffuse pain. A third type of fiber, A‐beta (Aß), is a fast, large‐diameter, myelinated fiber that carries action potentials from mechanoreceptors. Aß fibers are believed to modulate C and Að activity within the dorsal horn. There is a good deal of sensory overlap between these receptors, allowing a continuum of sensation [8]. The presynaptic terminals of Að and C fibres release a variety of pronociceptive substances into the synaptic cleft. C fiber presynaptic terminals are known to release glutamate which activates postsynaptic alpha‐amino‐3‐hydroxy‐5‐methyl‐4‐isoxazole propionic acid (AMPA) receptors; substance P (SP), which activates postsynaptic NK1 receptors; and calcitonin gene‐related peptide (CGRP), which activates postsynaptic CGRP receptors. In the spinal cord, the signal is either amplified or suppressed (modulation, phase 3) by excitatory or inhibitory interneurons that are continually bombarded with nociceptive input. Neurotransmitters of importance at this level include peptides (substance P, CGRP), amino acids (glutamate and aspartate), gamma‐aminobutyric acid (GABA), nitric oxide, prostaglandins (e.g. PGE2), adenosine triphosphate (ATP), endogenous opioids, monoamines (serotonin, norepinephrine), protons, neurokinin A, and others (see Figure 19.1) [9]. Modulation is an important component of maladaptive pain (described below). Projection (phase 4) of the signals continues their travel toward the brain through the spinal tracts. There is some controversy over which tracts are involved, but the spinothalamic, spinoreticular, spinomesencephalic, and spinohypothalamic tracts appear to be prominently involved [8,11]. Perception (phase 5) is the integration and processing of nociceptive information by the brain to recognize a stimulus as painful, and then to produce a response. The areas of the brain involved in perception are the periaqueductal gray area, reticular activating system, and thalamus. Behavioral changes may result and signals can be initiated that travel down the descending spinal tracts. These descending signals may be sent to a motor unit to evoke movement. Alternatively, the signals may synapse on inhibitory neurons in the dorsal horn of the spinal cord, decreasing or abolishing the noxious signals ascending to the brain. Loss or removal of this descending inhibitory signal is called disinhibition and can increase pain through decreased endogenous opioid release. The older pain classification of acute and chronic pain has been replaced by the classification of pain as either adaptive or maladaptive [4]. Adaptive pain (acute) is the normal pain response to tissue injury. It can be classified as inflammatory or nociceptive, with either being a normal response to tissue damage. Nociceptive pain is a transient response to a noxious stimulus [12]. These are typically small aches and pains that are relatively innocuous and that protect the body from the environment [4]. Inflammatory pain is spontaneous pain and can include hypersensitivity to pain [12]. It occurs in response to tissue damage and the resultant inflammation caused by tissue trauma, injury, and surgery. Inflammatory pain causes suffering, and it responds to treatment [12]. Maladaptive pain is more chronic in nature and occurs when adaptive pain is untreated or poorly managed. This type of pain is classified as neuropathic, functional, or central pain. Neuropathic pain is spontaneous pain or hypersensitivity to pain resulting from damage to or a lesion of the nervous system [12]. Functional pain is a hypersensitivity to pain resulting from abnormal processing of normal input [4,12]. Central pain is pain initiated or caused by a primary lesion or dysfunction in the central nervous system (CNS) [4,12]. The thalamus typically serves as a relay station for nerve impulses from the periphery to the cortex of the brain. If adaptive pain goes untreated, the thalamus can spontaneously generate a pain signal. Tissue damage leads to persistent and noxious stimulation of pain receptors. Damaged cells release inflammatory mediators (leukotrienes, prostaglandins, cytokines, protons) that attract inflammatory cells and the release of more chemical mediators. These mediators cause an increased sensitivity of the peripheral nociceptors by lowering their threshold for stimulation. Centrally, neurons in the dorsal horn become hyperexcitable and more sensitive to low‐intensity stimuli. This phenomenon leads to pain hypersensitivity. The result is one or more of the following: a typically nonpainful stimulus is now being perceived as painful (called allodynia); a perceived increase in pain intensity from the same stimulus is now occurring over time (called wind‐up pain); or there is an exaggerated response to a painful stimulus (called hyperalgesia). Preparing an effective pain management plan is a crucial component of the treatment of the small animal ICU patient. The choice of drug(s), route of administration, and anticipated response to therapy are based on the underlying disease process that is responsible for causing the pain and the anticipation of pain hypersensitivity. The experience of pain is unique among individuals, and analgesia that is adequate for one individual might not be adequate for another. The information from the patient history, physical, orthopedic and neurological examinations, and laboratory and imaging results will be important. The best analgesic should be chosen and administered by the most effective route. Close patient monitoring is required to determine the success of analgesic therapy and for early detection of any deleterious side‐effects of the drugs. Pain scales have been developed that illustrate and describe the psychological and behavioral activity and response to palpation, demonstrated at varying levels of pain intensity in the cat (Figure 19.2) and dog (Figure 19.3). The World Small Animal Veterinary Medical Association Global Pain Council has developed a set of guidelines to aid the veterinary team in the recognition, assessment, and treatment of pain in the dog and cat [13]. However, there is no specific behavior that is pathognomonic for pain, and behaviors at home may differ significantly from behavior in other environments. Severely injured or ill animals may not be able to express the typical behavioral pain responses due to their underlying condition. Therefore, the diagnostic information gathered must be used to identify any underlying problems that are likely to be causing pain and to recognize any signs attributable to pain exhibited by the patient. Figure 19.2 Colorado State University Feline Acute Pain Scale. Used with permission from Dr PW Hellyer, Colorado State University. Figure 19.3 Colorado State University Canine Acute Pain Score. Used with permission from Dr PW Hellyer, Colorado State University. The history begins with the signalment (age, sex, breed). Age‐related problems such as arthritis or severe periodontal disease are a potential cause of pain in older dogs and cats. There might be a breed disposition to potentially painful problems such as disk disease (Dachshund, Poodle, Basset Hound), orthopedic abnormalities (Rottweiler, Labrador Retriever, German Shepherd) or gastric distension (large breed dogs). Past medical problems can reveal conditions that might be associated with intermittent or low‐grade continuous pain, such as neuromuscular disease, orthopedic problems, severe skin disease, chronic wounds, chronic recurrent GI disease, or prostatitis. A list of prescribed and over‐the‐counter medications might reveal glucocorticosteroid or nonsteroidal antiinflammatory drugs (NSAIDs) that might be causing GI ulceration and pain. The presenting complaint may warrant immediate concern for the animal experiencing pain (such as trauma, lameness, swelling). However, it is frequently the description of the change in behavior of the animal that provides an alert to the presence of pain. Both dogs and cats may demonstrate hiding, lack of interactions or curiosity, anxiety, submission or guarding as a response to pain. Loss of appetite may be reported or the owner may complain about the pet showing aggression, attempts to escape confinement or restraint, or unusual grooming behavior (including self‐mutilation). Some animals become submissive or mentally depressed. Cats will often be lethargic and inappetent when painful. Loss of house training is not uncommon, with cats failing to use the litter box and dogs urinating or defecating in the house or cage. In extreme situations, patients will urinate or defecate where they are lying. Vocalizing is a nonspecific behavior, with pain as the first consideration, but other causes should be investigated (such as a full urinary bladder, the need to defecate, dysphoria from medications). Changes in body posture can include flatted ears, tail flicks, a stiff posture or gait, or guarding behavior in the cat. Dogs may lower their head and eyes, move their ears back or turn away. Altered facial expressions may also be associated with pain. In an animal that is reluctant to move due to pain, slight movement is often accompanied by other behavioral cues such as an abnormal gait, aggression, or vocalizing. Moderate to severe abdominal or thoracic pain is often demonstrated by a reluctance to lie down. They may stand in awkward positions (such as prayer position) for prolonged periods or may constantly reposition (stand‐sit‐lie down‐turn circles). Some animals will fall asleep in the stilted or sitting position, then begin to slump with the movement causing pain and abruptly waking to resume the original position. The physical examination begins during the recording of the history, when a distant exam is performed: the posture, behavior, and gait of the patient are observed for signs of painful behavior. The physical perfusion parameters (temperature, pulse rate and intensity, heart rate and rhythm, capillary refill time (CRT), and respiratory rate and effort) are then assessed. Tachycardia, tachypnea, hyperemic mucous membranes, rapid CRT, and bounding pulses could be due to either the compensatory stage of shock or to pain, each capable of eliciting a systemic sympathetic response. Physical evidence of poor peripheral perfusion seen in the later stages of shock (prolonged CRT, tachycardia (dogs), weak peripheral pulses, and pale mucous membranes) can mask the sympathetic response from pain. Therefore, analgesics become a vital component early in the shock resuscitation plan to reduce the contributions of pain to the sympathetic response (see Chapter 2). A careful head‐to‐tail examination is done, leaving manipulation of any potentially painful body regions to the latter portion of the exam. Adequate restraint is required and a muzzle or e‐collar is placed (when necessary) prior to manipulation of painful areas. The head is examined for symmetry and the cranial nerves are evaluated. Ptyalism can be a sign of pain in the cat and dog, especially with severe dental or other GI disease. Mydriasis may also be a sign of the sympathetic pain response. The hands are passed along all aspects of the body, feeling for any lumps, swelling, heat, sores or wounds that could be painful. Mild pressure is applied along the vertebral column, ribcage and down the limbs to assess for discomfort or other abnormalities. Abdominal palpation may induce splinting of the abdominal muscles, suggestive of generalized abdominal pain. Analgesics may be required to allow deep palpation of abdominal structures. Urinary bladder obstruction, peritonitis, pancreatitis, acute renal toxicity, abdominal hemorrhage, bowel ischemia, gastric or splenic torsion, gastric dilation, gallbladder inflammation or outflow obstruction, abdominal trauma, and mesenteric volvulus are causes of acute abdominal pain that warrant strong analgesic support for the patient. Neurological and orthopedic examination follows with careful manipulation of the head and neck, limbs, and joints. When testing the limb reflexes, it is important to differentiate reflex withdrawal (a reflex response) from withdrawal induced by pain (evidence of conscious recognition of pain). For example, in a nonambulatory dog with an L2–3 spinal lesion, the dog simply pulling his foot away when pinched does not indicate conscious awareness of a painful stimulus. He must also turn and look, cry out, growl, or in some way show he is aware of the painful stimulus. In equivocal cases, gently pinching an unaffected area (in the above example a digit on the thoracic limb would work well) and then comparing that response to a stimulus in the affected area can help to identify a stoic dog versus one that cannot sense the stimulus. Careful patient evaluation can fail to demonstrate a pain response in very stoic animals. When the patient has a condition expected to cause pain (such as trauma or surgery), treatment for the expected level of pain is very appropriate. A minimum database is provided through POC in‐hospital testing and should include the packed cell volume (PCV), total protein (TP), blood glucose, blood urea nitrogen (BUN), electrolyte panel, blood lactate, blood gas, coagulation profile, and urinalysis. Severe anemia, hypoglycemia, marked hyperglycemia, hypokalemia, significant azotemia, and severe acidosis can all cause weakness and mental depression, each capable of masking the physical signs of pain in the patient. Electrolyte disorders affecting sodium (Na), potassium (K), magnesium (Mg) or calcium (Ca) could impact nerve conduction and the neuromuscular response to pain. A high blood lactate suggests anaerobic metabolism, poor tissue oxygenation, and impaired perfusion. Analgesics are given and the blood lactate is monitored during fluid resuscitation for a declining trend as evidence of return of blood flow. Assessment of renal and GI function is necessary prior to administration of NSAIDs (see below). A complete blood count and serum biochemical profile provide data pertaining to possible causes of systemic problems that can cause pain. A high white blood cell count, a marked neutrophilic left shift, or a low white blood cell count can indicate severe tissue inflammation. The biochemical profile is assessed to evaluate for underlying disease and to assess the ability of the liver and kidneys to metabolize and excrete the prescribed analgesic drugs. Unfortunately, at this time no systematic relationship has been demonstrated between changes in plasma enzyme activities and established assessments of pain. The quantitation of catecholamines, cortisol, and ACTH has been used as a biochemical marker of pain in experimental animal models. However, the systolic, diastolic, and mean arterial blood pressure and heart rate have been suggested to be more sensitive than plasma ACTH or cortisol concentrations as indices of low‐grade pain [14]. Imaging provides a method for identifying a cause of recognized pain. Survey thoracic and abdominal radiographs provide the initial diagnostic imaging data once the patient has been stabilized and has received appropriate analgesics. The skeletal structures are closely examined for evidence of fracture, luxation, dislocation, inflammation, lysis or proliferative lesions that might be causing pain. Thoracic radiographs can demonstrate evidence of a mass lesion(s), pleural fluid or air, and rib or vertebral changes that might be associated with an observed pain response. Abdominal radiographs could show evidence of peritoneal fluid or air and provide an initial assessment of abdominal organ size, shape, and position. Abdominal and thoracic ultrasound provides a more in‐depth assessment of organ structure and can identify fluid pockets not seen on plain radiographs. The physical examination is the primary monitoring tool for assessing the presence and degree of pain. Pain scales (see Figures 19.2 and 19.3) are used to quantify the intensity of the pain and to follow the progress of pain control and patient comfort over time. These assessments rely on some objective observations but are still subject to interpretation, even between individuals. Use of the pain‐scoring system in a uniform and consistent manner by each patient care team member is essential to providing optimal pain control. Continued observation of the mental attitude, behavior, posture, and vocalization of the patient is imperative. The heart rate and blood pressure are physical parameters that reflect the demands on the sympathetic nervous system. Tachycardia and hypertension developing in an otherwise stable patient can be an early sign that the pain control is insufficient. At this time, the pain scale score is reassessed along with the analgesic drug selection, dose, and frequency of administration. The two key components to pain control in the acute setting are preemptive analgesia and multimodal therapy. Addressing these two components can reduce or prevent the conversion from adaptive pain to maladaptive pain. The old adage “an ounce of prevention is worth a pound of cure” is particularly applicable to preemptive pain control. Analgesia administered prior to inducing tissue trauma is associated with less wind‐up, a reduced overall need for analgesia, and a reduced need for rescue analgesia [4,15–18]. Multimodal analgesia is the practice of using multiple drugs in the pain control plan. Each drug should have a different mechanism of action and be complementary or synergistic. This technique allows use of lower doses of individual drugs to achieve pain control, reducing the incidence and severity of side‐effects of any one drug. Multiple drugs and routes of administration are available for use in critically ill patients. Common analgesic drugs used in the dog and cat are listed in Table 19.1 with dose, mechanism of action, and potential side‐effects. Analgesic drugs frequently combined for multimodal analgesia in the small animal ICU patient are listed in Table 19.2 along with dose and recommended route of administration. Severe adverse drug reactions (such as hypotension, dysphoria) can warrant altering the drug(s), dose, or mode of analgesia administered. Table 19.1 Selected analgesic drugs and doses for dogs and cats. CNS, central nervous system; CRI, constant rate infusion; CSF, cerebrospinal fluid; GI, gastrointestinal; IA, intraarticular; IM, intramuscular; IV, intravenous; NSAID, nonsteroidal antiinflammatory drug; OTM, oral transmucosal; PO, per os; SC, subcutaneous; SSRI, selective serotonin reuptake inhibitor. Table 19.2 Opioid‐lidocaine‐ketamine constant rate influsion for dogs and cats with moderate pain. Choose ONE of the opioid drugs, and combine with lidocaine and ketamine. Dexmedetomidine can be added for severely painful or anxious patients if cardiovascularly stable. Use the lowest rate that produces satisfactory analgesia to reduce adverse effects. Rate can be safely tripled for patients with severe pain. Analgesic drugs with different modes of action are used to target pain at different points along the pain pathway. Drug classifications that affect the peripheral nerves include NSAIDs, local anesthetics, and opioids. Drugs that work within the CNS (spinal cord and brain) include opioids, antidepressants, anticonvulsants, paracetamol (acetominophen), and local anesthetics. Opioids are generally considered as an important class of analgesics for small animal patients experiencing moderate to severe pain. Endogenous opioids (such as endorphins, enkephalins) are produced by the CNS and pituitary gland and released to inhibit the transmission of pain signals. There are naturally occurring G protein‐coupled opioid receptors (delta, kappa, mu, and nociception) located within both the central and peripheral nervous system and the GI tract. In the brain, stimulation of these receptors prevents the perception of pain. In the spinal cord, stimulation of opioid receptors prevents central sensitization, inhibits pain signal transmission, and inhibits neurotransmitter release. The efficacy of opioids in the peripheral tissues depends on the presence of inflammation, peripheral sensitization, and the presence or absence of hyperalgesia. Opioid drugs are classified by their selectivity and activity at the endogenous opioid receptors. There are three pharmacological classes of opioids: pure mu‐agonists, partial mu‐agonists, and the kappa‐agonist/mu‐antagonist. Pure mu‐agonists should be selected when treating moderate to severe pain. Morphine, hydromorphone, oxymorphone, fentanyl, and methadone are the pure mu‐agonists most commonly used in the dog and cat. These drugs provide superior analgesia at lower doses than the partial mu‐agonists (such as buprenorphine), and the kappa‐agonist/mu‐antagonists (such as butorphanol) at their higher doses. Naloxone is the reversal agent for the mu‐agonists. Buprenorphine is the most widely used partial mu‐agonist. It binds to the mu‐opioid receptor slowly (slow onset) but with a high affinity (long duration of action). Due to its partial activity, buprenorphine provides only moderate analgesia and variable sedation, with few adverse effects. When administered in cats, oral buprenorphine is absorbed transmucosally and provides good analgesia when dosed every six hours [19]. Butorphanol exhibits kappa‐agonist/mu‐antagonist activity at the opioid receptors. Receptor stimulation causes closing of influx membrane Ca channels and opening of membrane K channels, resulting in hyperpolarization of the cell membrane and suppression of transmission of action potentials in ascending pain pathways. At analgesic doses, butorphanol increases pulmonary arterial pressure and cardiac work. It also has antitussive effects. Sedation, nausea, and vomiting are possible side‐effects of butorphanol administration. Opioids often have a short half‐life in dogs and cats, and therefore require frequent administration. The occurrence of undesirable side‐effects after opioid administration can prompt the selection of a different opioid, addition of a sedative or switching to an analgesic with a different pharmacological action. An excitatory response to mu‐agonist administration is more common in the cat and relatively uncommon in the dog. The administration of a sedative, such as acepromazine, a benzodiazepine or dexmedetomidine, in combination with the opioid, can lessen the excitatory effects. This combination can result in the synergistic effect of neuroleptanalgesia (altered awareness and analgesia) and additional sedation. Alternatively, if the side‐effects of a mu‐agonist opioid are intolerable, the administration of a partial mu‐agonist or kappa‐agonist/mu‐antagonist (such as buprenorphine or butorphanol) can be used to partially reverse the effects of the original mu‐agonist drug. Naloxone can be given to fully reverse a pure mu‐agonist. However, naloxone will also eliminate any analgesic effects of the mu‐agonist opioid, requiring an alternate analgesic agent to be administered; the half‐life of naloxone is often shorter than that of the medication being reversed, so multiple doses may be required. Nonsteroidal antiinflammatory drugs are used as antiinflammatory, analgesic, and antipyretic agents. The pharmacological action is to block the arachidonic acid cascade by inhibiting the cyclooxygenase (COX) enzymes. This reduces the production of prostaglandins, an end‐product of the cascade and an inflammatory mediator that can cause an increase in the sensitivity of the peripheral nociceptors. There are three isoforms of COX enzymes: COX‐1, COX‐2, and COX‐3. Specific NSAIDs have been developed that selectively inhibit COX‐2 enzymes, with the intention of preserving the desired end‐product of COX‐1 activity, prostacyclin. Nonsteroidal antiinflammatory drugs can provide excellent analgesic and antipyretic effects in the ICU patient if the source of pain is inflammation. However, careful patient selection is mandatory. The animals must have normal tissue perfusion and hydration, and adequate renal function to receive an NSAID. Box 19.2 provides guidelines for minimizing the risk factors for adverse NSAID events.
Pain management
Introduction
Diagnostic procedures
History
Physical examination
Point of care (POC) testing
Clinicopathological testing
Imaging
Monitoring procedures
Pain management
Drug (duration)
Route
Dose for dog
Dose for cat
Notes
Pure mu‐agonist opioids
Fentanyl
IV CRI
2–5 µg/kg/h
2‐5 µg/kg/h
May cause urine retention; can be increased or decreased based on needs. Increase dose during painful procedures
Fentanyl (15–20 min)
IV
2–5 µg/kg
1–3 µg/kg
Fentanyl transdermal solution (Recuvyra) (3–4 d)
Proprietary intradermal injector
2.7 mg/kg
N/A
Special handling of drug and patient is required; refer to package insert
Hydromorphone
IV CRI
0.015–0.03 mg/kg/h
0.015–0.03 mg/kg/h
Hydromorphone (2–6 h)
SC, IM, IV
0.05–0.2 mg/kg
0.05–0.1 mg/kg
Methadone (2–6 h)
SC, IM, IV
0.2–1 mg/kg
0.1–0.5 mg/kg
Also NMDA receptor antagonist
Morphine
IV CRI
0.1–0.3 mg/kg/h
0.1–0.2 mg/kg/h
Morphine (2–4 h)
SC, IM, slow IV
0.5–1 mg/kg
0.2–0.5 mg/kg
May cause histamine release if given fast IV
Oxymorphone (2–4 h)
SC, IM, IV
0.05–0.4 mg/kg
0.02–0.1 mg/kg
Common side‐effects of pure mu‐agonist opioids include panting, nausea, sedation, mydriasis, hypothermia, hyperthermia, hyperexcitability, whining, defecation, constipation, bradycardia, respiratory depression; urinary retention has been reported with fentanyl.
Partial mu‐agonist opioids
Buprenorphine (6–12 h)
SC, IM, IV
5–20 µg/kg
5–20 µg/kg
Sedative effects apparent in about 15 minutes but requires 30–45 minutes for analgesia to occur
Buprenorphine (Simbadol) (24 h)
SC
N/A
0.24 mg/kg
Buprenorphine (6–8 h)
OTM
N/A
10–20 µg/kg
OTM bioavailability in dogs is only about 5% and not recommended
Partial mu‐agonists will antagonize the pure mu‐agonists. IM may be more painful than other agents. Common side‐effects include respiratory depression (rare and typically at high doses). Dogs may show hypersalivation, bradycardia, hypothermia, agitation, dehydration, and miosis. Tachycardia, vomiting, and hypertension have rarely been reported. In cats mydriasis and behavioral effects (e.g. excessive purring, pacing, rolling, etc.) have been reported. Vomiting and hypothermia are rare.
Agonist‐antagonist opioids
Butorphanol (1–4 h)
SC, IM, IV
0.1–0.4 mg/kg
0.1–0.4 mg/kg
Butorphanol
IV CRI
0.03–0.4 mg/kg/h
0.03–0.4 mg/kg/h
Loading dose 0.1 mg/kg IV
Much better sedative than analgesic. Dogs with MDR1 mutation may have more pronounced sedation, and sedation may persist longer in these dogs. Use with caution in animals with head trauma, increased cerebrospinal fluid pressure. Adverse effects reported include sedation, excitement, respiratory depression, ataxia, anorexia, diarrhea (rare).
Alpha‐2‐adrenergic agonists
Dexmedetomidine
IM, IV
125–250 µg/m2
150–300 µg/m2
Dexmedetomidine
IV CRI
0.5–2 µg/kg/h
1–2 µg/kg/h
Do not use in pregnant animals, cardiovascular disease, respiratory disorders, liver or kidney disease, patients in shock, patients with severe debilitation, or severe stress due to extreme heat, cold, or fatigue. The UK label states not for use in puppies <6 months or kittens <5 months of age. Most adverse effects are extension of pharmacological action: bradycardia, vasoconstriction, muscle tremors, transient hypertension, reduced tear production, occasional atrioventricular block, decreased respiration, hypothermia, urination, vomiting, hyperglycemia. Rarely see prolonged sedation, paradoxical excitation, hypersensitivity, pulmonary edema, apnea, and death from circulatory failure. Adverse effects are generally reversed by administration of reversal agent (atipamezole), which also reverses analgesic effects. Concurrent use of atropine with bradycardia is not recommended.
Local anesthetics
Bupivacaine
Local infiltration, nerve blocks, IA
Up to 2 mg/kg total dose
Up to 1 mg/kg total dose
Lidocaine
Local infiltration, nerve blocks, IA
Up to 12 mg/kg total dose
Up to 6 mg/kg total dose
Lidocaine
IV CRI
30–80 µg/kg/min
(1.8–5 mg/kg/h)
Not recommended
1–2 mg/kg IV loading dose; do not use the product containing epinephrine IV
Lidocaine
Transdermal patch
Apply to affected area over intact skin
Cats are more sensitive to the cardiodepressant and CNS effects of lidocaine so use with caution in cats. Most common adverse effects are dose related and mild. CNS signs (drowsiness, depression, ataxia, muscle tremors), nausea and vomiting (usually transient). At high doses adverse cardiac effects and seizures can occur. Use with caution in patients with liver disease, congestive heart failure, shock, hypovolemia, severe respiratory depression, marked hypoxia, bradycardia, or incomplete heart block.
NMDA receptor antagonists
Ketamine
Intermittent IV
0.5 mg/kg
0.5 mg/kg
NMDA receptor antagonism
Ketamine
IV CRI
0.6 mg/kg/h during surgery
0.12 mg/kg/h for next 24 h
0.6 mg/kg/h during surgery
0.12 mg/kg/h for next 24 h
NMDA receptor antagonism
Contraindicated in patients with significant hypertension, heart failure, dogs with liver failure, cats with kidney failure. Does not provide muscle relaxation. Increases CSF pressure at anesthetic doses (unlikely at analgesic doses). Common adverse effects include hypertension, hypersalivation, respiratory depression, hyperthermia, vomiting, vocalization, and prolonged and erratic recovery, dyspnea, seizures, muscle tremors, opisthotonus. These effects are extremely rare at analgesic doses listed above.
Miscellaneous oral medications
Amantadine
PO
2–5 mg/kg q 24 h
2–5 mg/kg q 24 h
NMDA receptor antagonism
Amitriptyline
PO
1–2 mg/kg q 12–24 h
0.5–1 mg/kg q 12 h
SSRI; may be useful for chronic neuropathic pain
Dextromethorphan
PO
0.5–2 mg/kg q 24 h
NMDA Receptor antagonism
Gabapentin
PO
3–10 mg/kg q 12–24 h
1.25–5 mg/kg q 24 h
Decrease dose slowly to avoid seizures; some liquid formulations contain xylitol (toxic to dogs)
Tramadol
PO
2–10 mg/kg q 8–24 h
1–5 mg/kg q 12–24 h
Useful for low‐grade pain. Efficacy is questionable in cats
Amantadine has a narrow therapeutic window, and both dogs and cats are quite sensitive to overdose. May need to be compounded to provide safe appropriate dose. Adverse effects in dogs include behavioral changes, flatulence, and loose stools, especially early in therapy; limited experience/data in cats. Amitriptyline may reduce the seizure threshold in dogs and cats. Sedation and anticholinergic effects are the most common adverse effects. Taper slowly when discontinuing this medication. Severe adverse effects seen with severe overdose include cardiorespiratory collapse and cardiac arrhythmias. Common milder adverse effects (usually associated with overdose) include drowsiness, dry mouth, constipation, abnormal bleeding, seizures, tachycardia, fever. Gabapentin use with caution in animals with kidney failure; some human liquid formulations contain xylitol – do not use these formulations in dogs. Sedation and ataxia are most common adverse effects. Tramadol most common adverse effects are excessive sedation, agitation, anxiety, tremors, inappetance, vomiting, constipation, or diarrhea.
NSAIDs
Acetaminophen
PO
10–15 mg/kg PO q 8 h; consider q 12 h dosing if using longer than 5 days
DO NOT USE IN CATS
Hepatotoxicity and methemoglobinemia in cats; liver and renal function should be assessed beforehand
Carprofen
SC, PO
2–4 mg/kg (max dose 4.4 mg/kg/day)
(UK label; extralabel in USA) 4 mg/kg SC or IM once
Mild GI effects; rarely severe hepatic failure
Deracoxib
PO
1–2 mg/kg q 24 h
Also labeled 3–4 mg/kg PO q 24 h for postop pain
Etodolac
PO, SC
5–15 mg/kg q 24 h
Meloxicam
IV, SC, PO, OTM
0.1–0.2 mg/kg
0.1–0.2 mg/kg injection (Metacam) ONCE
Robenacoxib
PO, SC
1 mg/kg PO2 mg/kg SC
Maximum 3 days
Tepoxalin
PO
10 mg/kg q 24 h
Do not mix NSAIDs with steroid drugs or exceed dose – GI perforation is highly likely. Vomiting is the most common sign associated with NSAID‐associated GI perforation. Most common adverse effects include vomiting, diarrhea, kidney injury, impaired platelet activity, and CNS depression. Liver and kidney function should be assessed prior to initiating therapy, and regularly with prolonged (>7–10 days) therapy. For all NSAIDs, use the minimum effective dose for long‐term therapy.
Reversal agents
Naloxone
IM, IV, SC
0.002–0.1 mg/kg
0.002–0.1 mg/kg
Reversal for opioid drugs
Atipamezole
IM, SC (IV in emergency only)
0.05–0.2 mg/kg
0.05–0.2 mg/kg
Reverses alpha‐adrenergic agonist drugs
Drug
Dogs
Cats
Loading dose
Fentanyl
‐OR‐
Hydromorphone
‐OR‐
Morphine
3 µg/kg/h
0.13 mg/kg/h
0.1 mg/kg/h
3 µg/kg/h
0.006 mg/kg/h
0.1 mg/kg/h
5 µg/kg
0.05 mg/kg
0.3 mg/kg
Lidocaine
1.8 mg/kg/h
Not recommended
2 mg/kg (dogs only)
Ketamine
0.6 mg/kg/h
0.6 mg/kg/h
1 mg/kg
Dexmedetomidine
1 µg/kg/h
1 µg/kg/h
100 µg/m2
Analgesic drugs
Opioids
Nonsteroidal antiinflammatory drugs (NSAIDS)
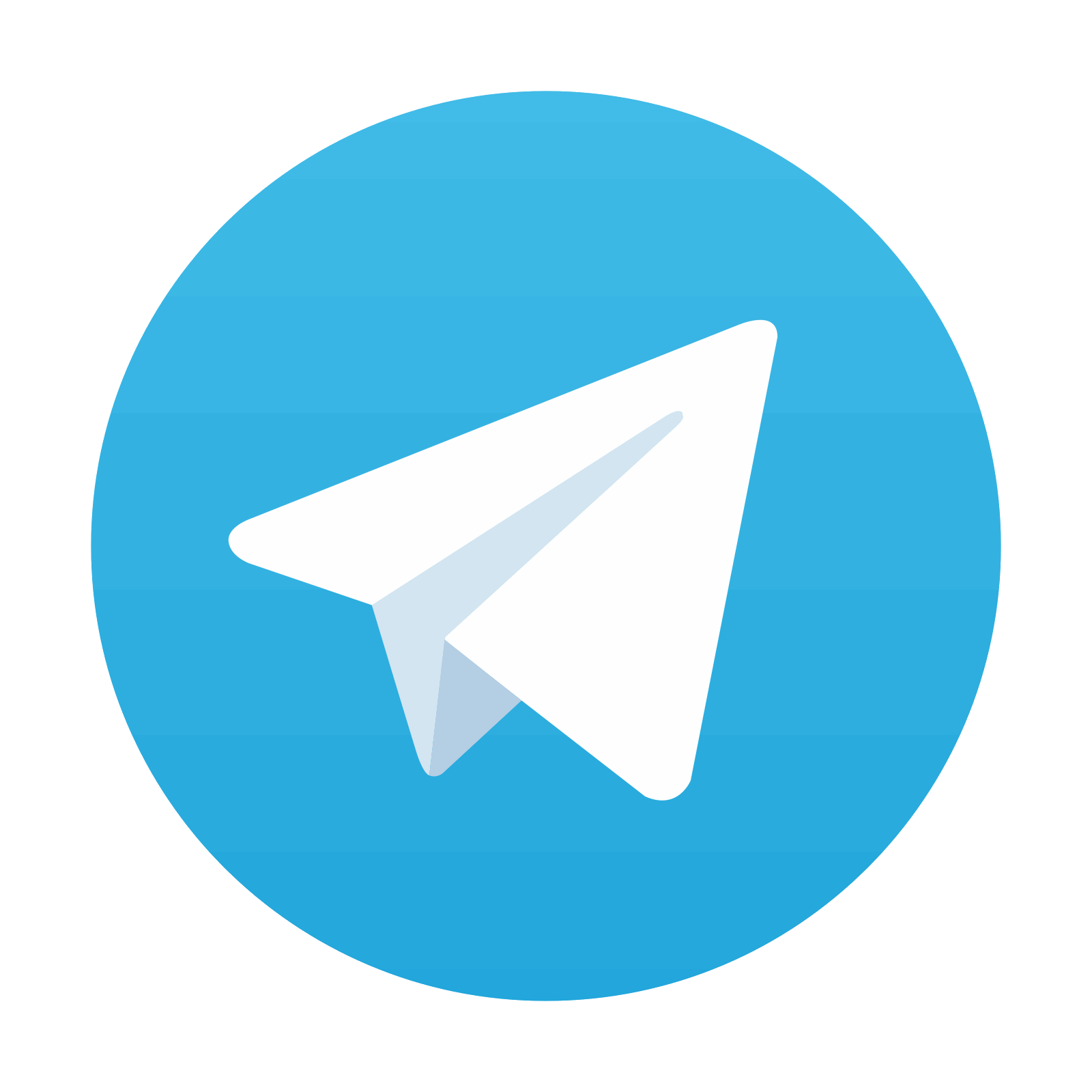
Stay updated, free articles. Join our Telegram channel

Full access? Get Clinical Tree
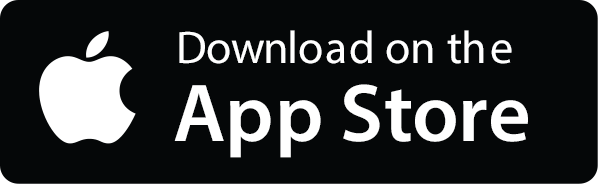
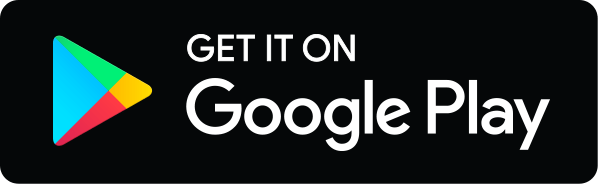