CHAPTER 18 Dawn Merton Boothe College of Veterinary Medicine, Auburn University, Auburn, Alabama The goal of drug therapy is to induce in the patient a desired pharmacological response for an adequate period of time while avoiding adverse drug events. Reaching this goal requires the administration of the right drug (drug selection), in the right amount (dosing regimen), at the right time (dosing interval). The magnitude of response to any drug is determined by the concentration of the drug and the affinity of the drug for the targeted receptors. The desired response could be due to the original drug that was administered, the metabolites of the administered drug or a combination of effects. This includes “prodrugs” which are drugs that must be activated prior to eliciting the intended response. Dosing regimens are composed of a route, dose (such as mg/kg) and interval (such as every eight hours: q 8 h). The goal is to achieve and maintain the desired response throughout the dosing interval. The route of administration of a drug is mandated by the drug preparation and by patient needs. Therefore, it is the drug dose and interval that are most often manipulated to achieve the goals of drug therapy. The therapeutic range of a particular drug is characterized by a plasma drug concentration (PDC). The Cmin is the minimum PDC below which therapeutic failure is likely to occur. The Cmax is the maximum PDC above which side‐effects, adverse events or toxicities are more likely to occur. When deciding to initiate drug therapy, 10 basic questions should be considered (Box 18.1). The selection of the right drug for a critical patient requires knowledge of the effect that factors specific to the patient (Table 18.1) can have on drug absorption, distribution, metabolism, and excretion (ADME). To achieve the optimal drug response with minimal negative side‐effects, it is necessary to carefully select the drug(s) and give special consideration to drug dose and interval of administration for each drug prescribed. Table 18.1 Patient factors affecting drug pharmacodynamics and pharmacokinetics. BSA, body surface area; CNS, central nervous system; NSAID, nonsteroidal antiinflammatory drug; PDC, plasma drug concentration; sAME, S‐adenosyl methionine; Vd, volume of distribution. Benefit versus risk should be the most important determinant of drug selection. The objective is to assess whether the drug is necessary and beneficial and determine if the effects are measurable and safe. Unfortunately, the most effective drug can also be associated with the greatest risk of adverse effects. The individual characteristics of a drug should be evaluated during the drug selection process. The pharmacodynamics of a drug describes the concentration and subsequent pharmacological effects of that drug. Drug pharmacokinetics describes the properties responsible for maintaining effective drug concentrations, to include bioavailability, absorption, distribution, metabolism, volume of distribution (Vd), half‐life, and elimination. The ideal drug should incorporate one or more of the following pharmacological traits: While receptor specific drugs are generally associated with fewer adversities, the “selectivity” may be lost with overdosing. Similar changes in magnitude and direction should occur between the drug concentrations in the plasma and drug concentrations at the targeted tissue or receptor. This is determined by appropriate drug dosing and by drug absorption and distribution. Selection of antimicrobials justifies special consideration. The initial drug is typically chosen empirically, based on common etiological agents and drug qualities. Little information exists to support empirical selection of antimicrobials in the critically ill small animal patient, yet these patients can be the most vulnerable to therapeutic failure. Unfortunately, the advent of antimicrobial resistance is limiting efficacy of many commonly prescribed antimicrobial drugs. It is critical that the antibiotic choice is made with knowledge of the most current antibiograms that have been prepared specifically for the veterinary hospital or geographical region. An estimation of the likelihood for resistance can be determined. The procedures for hospital antibiotic surveillance and compilation of an antibiogram can be found in Chapter 14. Many commercial veterinary laboratories can provide a current antibiogram for the geographical region or for the specific veterinary hospital. Both patient factors and drug factors (ADME) will have an important impact on drug disposition or behavior. Patient factors (see Table 18.1) will include normal physiological differences as well as pathological factors caused by the disease. Drug factors include a wide variety of drug–drug (and potentially drug–diet) interactions that may alter the response of the individual patient. Significant interactions have been reported between drugs and some dietary supplements in human patients. A review of all current medications and supplements becomes important to determine any potential of negative drug interactions and to ascertain whether any new clinical signs could be the result of one or more of the drugs. The drug concentration at the site of action generally parallels PDC, and is determined by four simultaneously occurring drug activities: absorption, distribution, metabolism, and excretion. Figure 18.1 provides a schematic demonstrating the general pathways for drug ADME. Figure 18.1 Schematic of drug movements from absorption through elimination. Response to a drug reflects the extend of drug–receptor interaction and thus concentration of drug at the tissue. Tissue drug concentrations generally parallel plasma concentrations, which in turn reflect four simultaneous drug movements. Drug absorption depends on the route of administration, with intravenous (IV) providing 100% drug bioavailability. Oral absorption is impacted primarily by gastrointestinal pH, motility, surface area, and epithelial permeability. First‐pass metabolism by the liver decreases oral bioavailability. Factors that affect drug distribution include protein binding, regional blood flow, transport proteins, tissue‐drug binding, and body compartment size. Elimination of the plasma drug concentration can be through hepatic metabolism for lipid‐soluble drugs, which undergo Phase I and possibly Phase II metabolism, or renal excretion of water‐soluble drugs. Lipid‐soluble drugs also may be excreted in the bile, some of which will undergo enterohepatic circulation. Each of the drug movements is impacted by the concentration of diffusible drug and thus drug lipophilicity, molecular weight, and drug pKa. GI, gastrointestinal; IM, intramuscular; SC, subcutaneous. The most common method of drug movement is by passive diffusion and as such, the single most important determinant of how much drug moves is the concentration of diffusible drug. Water‐soluble drugs primarily distribute to the extracellular fluid (ECF) while lipid‐soluble drugs tend to penetrate cell membranes. The extent of drug movement depends on the size of the body compartments to which the drug is being distributed. The apparent volume of tissue distribution (Vd) is the volume of tissue which dilutes the drug and is calculated by Vd = dose/PDC. Small, lipid‐soluble and unionized drug molecules are more likely to move beyond cell membranes or other barriers. A drug that is ionized will be trapped at the site. In general, the closer the bodily fluid or tissue pH is to the pKa of the drug (equal parts ionized versus unionized), the more diffusible the drug. Although passive drug movement predominates, the importance of active transport through efflux or influx proteins is being recognized. These transport pumps generally move weak acids, weak bases or organic (nonionizable) drugs against a concentration gradient. Their role in renal and biliary excretion is well recognized. These pumps can markedly impact drug absorption and prevent effective drug penetration at isolated sites, such as the brain, cerebral spinal fluid, eye, prostate, and testicles, Absorption can be described by the rate and extent of the drug being absorbed and the systemic bioavailability of the drug. The bioavailability is the percent or fraction (F) of a drug dose that reaches systemic circulation. By definition, a drug given intravenously is 100% bioavailable (F = 1). The difference between an IV dose and a non‐IV dose is represented by the formula: Dose non‐IV = dose IV/F. Intravenous administration is common in the critically ill patient. Although the risk of an exaggerated response might be increased, an advantage to IV administration is the removal of the effect of disease, diet or other drugs on drug absorption. Many factors influence oral drug absorption. Because of the large surface area in the small intestines, most oral drugs are absorbed from that location. The rate and extent of oral drug absorption are influenced primarily by environmental pH (generally acidic but becoming more alkaline distally), motility, epithelial permeability, surface area, blood flow, and bile salt production. Transport proteins (P‐glycoprotein in particular) and drug‐metabolizing enzymes in intestinal epithelial cells can markedly contribute to differential drug absorption. Even drugs that are well absorbed after oral administration may not have a high systemic bioavailability. First‐pass metabolism occurs when much of the absorbed drug is extracted by the portal circulation. For these first‐pass drugs, the oral dose is generally much higher than the parenteral dose. Alternate routes, including transmucosal or rectal administration, might be considered for such drugs. Further, systemic bioavailability of these drugs is markedly influenced by changes in portal blood flow. Parenteral (subcutaneous, intramuscular) absorption is influenced primarily by surface area and regional blood flow. Many drugs are modified to prolong parenteral absorption by the addition of salts or esters that will release the drug. The parenteral and oral administration of drugs should be avoided in critical patients until regional perfusion is restored. Transdermal administration deserves special consideration. The stratum corneum is a major barrier to absorption of all except the most lipophilic of drugs. FDA‐approved special delivery systems such as transdermal patches or liquids may effectively deliver drug by increasing epithelial permeability by altering temperature or hydration. However, caution is recommended when using compounded complex delivery systems since there may be limited data to support the safety, efficacy or quality of such preparations. Distribution is one of two drug movements that eliminates drug from plasma. Distribution includes movements of the drug from plasma into tissues and back again, with elimination from the body the final movement. Factors that determine distribution include regional blood flow, tissue‐drug binding, and transport proteins. Protein binding, principally to albumin, causes a lipid‐soluble drug to be sufficiently water soluble, allowing the drug to move through the body. However, while protein bound, the drug is inactive. The amount of tissue to which an IV administered drug is distributed is the main determinant of PDC. The Vd simplistically is the volume of tissue which dilutes the drug and can be calculated by Vd = dose/PDC. As such, if a target PDC and the Vd of a drug are known, the dose needed to achieve the desired PDC (and thus the desired response) can be calculated by Dose (mg/kg) = PDC (mg/L) × Vd (L/kg). Since dose is directly proportional to Vd, an increase in the Vd necessitates that the dose is also increased to maintain the same PDC. Likewise, if the Vd decreases (such as dehydration), the PDC will increase, possibly resulting in toxicity. When the drug is given by a nonintravenous route, then the dose must be further modified by F (Dose non‐IV = dose IV/F). All drugs initially distribute to plasma (about 0.05% of body weight). Drugs that are tightly and significantly (>80%) bound to plasma proteins generally remain in the plasma and have a Vd ≤0.1 L/kg. The fraction of unbound drug can be easily influenced by changes in protein concentration and by competition with other drugs or compounds; however, this unbound fraction may be more rapidly cleared, decreasing the risk of adverse reaction. Drug solubility affects the distribution of the drug. Water‐soluble drugs primarily distribute to the ECF (20–30% of body weight,) resulting in a Vd generally between 0.2 and 0.3 L/kg. Lipid‐soluble drugs tend to penetrate cell membranes, being distributed to total body water (60% or more of body weight) with a Vd ≥0.6 L/kg. Drugs that are ion trapped, bound to tissue proteins or accumulate for other reasons are removed from plasma and are characterized by higher PDC. This often results in Vd >1 L/kg. Because distribution of a drug can remove it from the organs of clearance, the half‐life of these drugs tends to be long, and the time to steady state, the point at which both safety and efficacy can be assessed, will also be prolonged. Once an equilibrium is reached between drug distribution and elimination, the decline in PDC reflects only drug elimination from the body. Metabolism is the means by which the body converts a lipid‐soluble drug, which will be passively reabsorbed as urine is concentrated in the renal tubule, into a water‐soluble drug, which will not be passively reabsorbed and will subsequently be excreted. Most drug metabolism occurs in the liver. Hepatic clearance of a drug is affected by hepatic blood flow and the intrinsic metabolism and protein binding of the drug. The influence of each of these varies with the drug, and determines whether the drug metabolism is “flow‐limited” or “capacity‐limited.” For “flow‐limited” drugs (such as lidocaine, most beta‐adrenergic drugs, most opioids, benzodiazepines), clearance is dependent only on the rate of delivery to the liver and thus changes directly and proportionately with changes in hepatic blood flow. Orally administered drugs enter the portal circulation and undergo “first‐pass metabolism.” These drugs are characterized by a high hepatic extraction (>70% with each passage) and are not impacted by protein binding. Clearance of “capacity‐limited” drugs (most nonsteroidal antiinflammatories, diazepam, prednisolone, phenylbutazone, phenytoin, theophylline, cimetidine, and antipyrine) is limited by the intrinsic metabolic capacity of the liver. Protein binding can prevent the clearance of capacity‐limited drugs. Hepatic drug metabolism is generally accomplished in two phases. Phase I metabolism enzymes (primarily cytochrome p450; CYP450) most often inactivate a drug. However, drugs may also be converted to an active metabolite with equal or less activity. Prodrugs must be converted to an active metabolite (such as enrofloxacin converted to ciprofloxacin; prednisone converted to prednisolone). Phase I metabolism also can metabolize a drug to a toxic metabolite, with such drugs increasing the risk of hepatotoxicity. Toxic metabolites are also common sources of haptens, molecules that associate with tissues and cause an allergic response. Phase I metabolism is often, but not always, a prelude to Phase II metabolism. Phase II hepatic metabolism results in the addition of a large water‐soluble molecule to the drug, generally rendering the drug inactive (or nontoxic), and facilitating its renal excretion (or, less commonly, biliary excretion). As such, Phase II metabolism is often protective in nature. Common conjugates include glucuronide (some deficiencies in the cat) and glutathione, the latter being particularly effective in scavenging toxic drug metabolites. However, the liver’s protective Phase II metabolic capacity is both dose and duration dependent. Acetylation often produces active metabolites and is a reaction in which the dog is deficient. The metabolic pathway of each drug varies markedly with the chemistry of the drug and the species of the patient. Over 20 superfamilies of CYP450 have been identified. Species differences are marked, with many drugs able to induce or inhibit many of these enzymes. Excretion is the drug movement that clears drug or its metabolites from the body. The kidney is the most important organ of excretion. All drugs undergo glomerular filtration; however, the extent of excretion of that drug in urine depends on how much is passively resorbed, which in turn depends on lipid solubility, ionization, and molecular weight. In addition to glomerular filtration, which is a passive process, many drugs are actively secreted into the urine in the proximal tubules by way of transport proteins. All drug movements in the kidney are influenced by renal blood flow. Therefore, any disease or drug that affects renal blood flow will have an impact on renal drug clearance. The extent of renal clearance of a drug can be predicted based on creatinine clearance or serum creatinine, which can be used to modify dosing regimens (Box 18.2). However, urine pH can have a profound effect on the extent of renal excretion of a drug. In contrast to renal clearance, biliary clearance is slow. Generally, very large drugs are excreted in the bile (such as macrolides, cyclosporine).
Drug selection and dosing regimens
Introduction
Factor
Effect
Examples of drugs affected
Adjustment
Fluid balance
Increased fluid balance: IV fluid administration, fluid retention (except for third body compartments)
Poor peripheral tissue perfusion:dehydration, hypovolemia, vasoconstrictive drugs
Compartmentalized third body fluid space: ascites, pleural effusion
Plasma and tissue drug concentrations
Half‐life (this may be offset by clearance of renally eliminated drugs
Absorption SC, IM (primarily rate)
PDC due to smaller volume of distribution
Distribution to brain and heart
Decreased renal clearance until volume replacement occurs) (prolonged)
or no change in half‐life
Impact depends on distribution of drug: decreased volume of distribution if a water‐soluble drug. If lipid soluble, dose on total body weight.
Binding to effluxed proteins
Water‐soluble antibiotics: aminoglycosides, beta‐lactams
Any drug, but the risk is greater with water‐soluble drugs. Care with cardioactive and CNS active drugs in particular
Increase dose to enhance efficacy
Longer dosing interval to avoid adverse effects
Anticipate longer onset to response
Decrease dose
particularly for CNS or cardioactive drug until volume replaced (this may also shorten half‐life)
Prolong interval in anticipation of longer duration of effect, particularly in patients with altered renal function
Half‐life may not change even if profound changes in distribution or clearance
Dose based on lean body weight or BSA
Hypoalbuminemia
Renal clearance if organ function normal (shorter half‐life)
Free drug available at receptor (longer half‐life)
Antimicrobials: cefovecin, doxcycyline
Imidazole antifungals
All nonsteroidal antiinflammatories
Decrease dose if abnormal clearance; prolong interval, particularly if abnormal clearance
Inflammatory proteins/acute‐phase proteins
binding by basic drugs; will facilitate clearance of flow‐limited drugs
Lidocaine, opioids
May need to alter dose
Renal disease
Uremic toxins
Abnormal proximal tubular secretion Renal blood flow
Altered tissue receptors; Competition for drug binding sites; change conformation/affinity for binding protein
Excretion/
PDC; abnormal transport of drugs and organic acids
Excretion; abnormal transport of drugs and bases
Renal extraction
NSAIDS, selected penicillins, furosemide, anticonvulsants
Penicillins, cephalosporins, NSAIDs, sulfonamides, several diuretics
Cimetidine, procainamide, morphine derivatives
Penicillins, sulfates, glucuronide conjugates
Reduce dose using creatinine clearance
Adjust dosing interval for drugs that accumulate; adjust either interval or dose for drugs that do not accumulate
Adjust dosing interval for drugs that accumulate; adjust either interval or dose for drugs that do not accumulate
Liver disease
Hepatoportal/intrahepatic shunting
Chronic liver disease
Flow‐dependent metabolism
Phase I and II drug metabolism/clearance
Propranolol, verapamil, prazosin, morphine derivatives
Diazepam, prednisolone, phenylbutazone, phenytoin, theophylline, cimetidine, antipyrine
Reduce dose
Addition of liver protective drugs may be beneficial: N‐acetylcysteine, sAME, milk thistle
Cholestasis
Activity/amount p450;
drug elimination in bile
Doxycycline, clindamycin, digitoxin, naproxin
Reduce dose
Sepsis
Increased capillary permeability
Hyperperfusion
Vd
Renal and hepatic clearance of flow‐
dependent drugs Rate of hepatic metabolism;
PDC
Aminoglycoside
Increase dose
Increase dose
Brain trauma
Rate of hepatic metabolism
May need to alter dose
Biliary tract disease
Bilirubinemia competes for albumin binding site
May need to alter dose
Electrolyte, acid–base disorders
Δ pH
Altered receptor sensitivity to drugs
Δ drug distribution
Hyperkalemia effect on digitalis, quinidine, procainamide
Delay drug administration until correction or consider altered dose
Gastrointestinal disorders
Abnormal mucosal transport, motility, blood flow, efflux of proteins
Poor absorption of oral drugs; drug binding to effluxed proteins
Use parenteral routes of administration
Nutritional status
Obesity
Poor enteral feeding;
enterocyte atrophy w/in 3 days
Oral feeding
Fat distribution of lipophilic drugs
Poor absorption; abnormal hepatic drug metabolism
Diet impacts on absorption of oral drugs
Fever
Poor oral absorption
Amoxicillin
Avoid oral drugs
Cardiovascular disease
Na+, H2O retention
Hypertension/
vasoconstrictionCardiac output
Δ Vd/drug distribution Hepatic clearance
Redistribute blood flow; drug to brain & heart;
absorption SC, IM, oral;
clearance of flow‐dependent drugs
Lidocaine, digoxin
Species
Feline
Deficiency of some glucuronyl transferases in Phase II metabolism; deficient in many phase I metabolism enzymes;
different responses to some drugs
Opioids, dopamine, fluoroquinolones
Avoid drugs that form toxic metabolites; closely adhere to drug recommendation for the species
Drug selection
Drug disposition
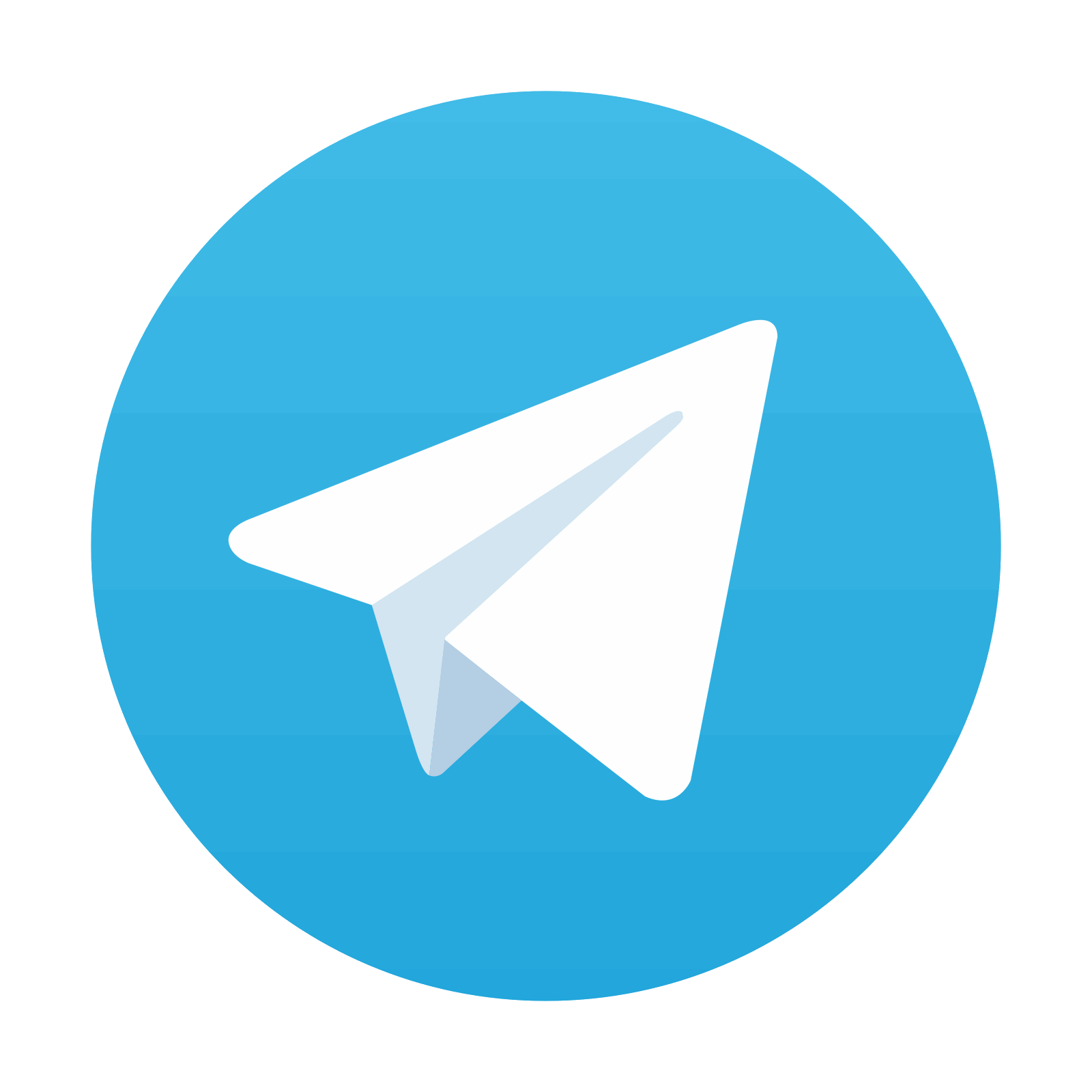
Stay updated, free articles. Join our Telegram channel

Full access? Get Clinical Tree
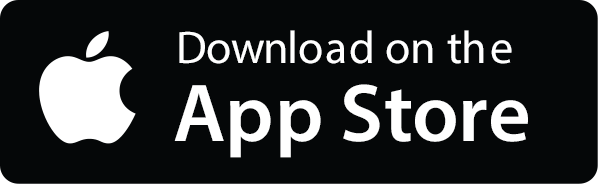
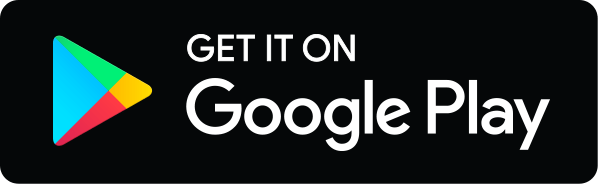