CHAPTER 14 Carol E. Haak Milwaukee, Wisconsin Life or death in the critically ill small animal patient is often the result of the host’s ability to mount a rapid and aggressive immune response. Both primary pathogens and secondary invaders can cause disease. Etiologies include bacteria, viruses, rickettsia, fungi, protozoa, parasites, and foreign material. The primary immune response should occur at the first exposure of the tissues or blood to the pathogen. A schematic of the basic mechanisms involved in this primary immune response (both innate and acquired) is illustrated in Figure 14.1. Figure 14.1 Schematic of the primary immune response to antigens or injury. The innate immune system is the first line of defense against pathogens. Efficacy does not require previous exposure to a specific invader. This nonspecific immune response includes physicochemical barriers, molecules normally present in body fluids (such as lysozyme, complement, antiproteases) and phagocytic and cytotoxic cells (such as PMNs, macrophages, NK cells, mast cells). This first‐line response protects the host from many extracellular organisms, but not all intracellular organisms (protozoa, viruses, certain bacteria). The acquired immune system is specific and has immunological memory through dedicated immune cells (lymphoid cells). Antibodies and immunoglobulins are produced that specifically counteract the antigens (viral, bacterial, fungal, foreign bodies). The T‐ and B‐cells recognize the antigen, proliferate in response to it, and migrate back to the site of injury. There they release cytokines that attract effector cells (cytotoxic T‐cells, activated macrophages, and committed B‐cells). Cytokines include interferons, interleukins, colony stimulating factors, and tumor necrosis factors. Antigen specificity is mediated by lymphocytes. This is the most important aspect of the acquired immune system. T‐cells typically respond to surface‐bound antigens (usually cell associated) and the B‐cells typically respond to soluble, extracellular antigens. The immune response is called “humoral” when antibodies are involved in removing antigen and “cell mediated” when T‐cells and macrophages are involved. The first immune response is dictated by the innate portion of the immune system. This response is nonspecific and does not require previous exposure to the antigen. The skin, gastrointestinal (GI) mucosal barrier, and mucous membranes form some of the innate physiochemical barriers to antigen invasion. Chemicals such as lysozymes, antiproteases, and complement present in bodily fluids can block replication or lyze the outer membranes of invading organisms. Phagocytic and cytotoxic myeloid cells including polymorphonuclear cells (PMNs) or neutrophils, macrophages, natural killer (NK) cells, and mast cells are recruited to the area of antigen deposition to destroy the pathogen. This approach can be very effective against many extracellular viruses and bacteria. However, many extracellular and most intracellular pathogens require a more sophisticated defense. After the initial innate response comes the acquired portion of the immune response. This is antigen specific and creates immunological memory through dedicated lymphoid cells. T‐ and B‐ lymphocytes are exposed to antigen, then proliferate and migrate back to the site of injury. Cytokines released attracts other effector cells. Activated B‐cells will synthesize and release immunoglobulins. Immunoglobulins (circulating) become bound to cell surfaces (antibodies), thus marking invaders and activating complement. Thus myeloid cells are recruited and the antigen is then neutralized or destroyed. The immune system of the ICU patient is constantly challenged by a multitude of internal and external factors. It is important to consider patient‐specific, disease‐specific, and hospital‐specific factors in the critically ill patient. Most commonly, we consider disease‐specific factors in our patients: open fracture, wounds, infectious agent, disease‐specific immunosuppression, chemotherapeutics, etc. However, patient‐specific factors such as retroviral status, GI function, risk of GI bacterial translocation, co‐infection due to aspiration, malnutrition, immune dysfunction or drug suppression often make a significant contribution to morbidity and mortality. At the other end of the spectrum, an abnormally active or improperly stimulated immune system may result in autoimmune or allergic disorders that can be equally life threatening. Additional factors are introduced in a hospital environment such as contamination of wounds or fractures, indwelling medical devices (IV and urinary catheters, suture material, implants), and nosocomial infections, which must all be considered. All of these may result in activation of the systemic inflammatory response syndrome (SIRS), multiple organ dysfunction syndrome (MODS), and death (Figure 14.2). Figure 14.2 Patient‐, drug‐, and organism‐specific considerations in antibiotic selection. CNS, central nervous system; GI, gastrointestinal; MBC, minimum bactericidal concentration; MIC, minimum inhibitory concentration; MPC, mutant prevention concentration. Source: Adapted from Giguère S, Prescott JF, Dowling PM. Principles of antimicrobial drug selection and use. In: Antimicrobial Therapy in Veterinary Medicine, 5th edn. Ames: John Wiley & Sons, 2013 [1]. Used with permission of Wiley. When a bacterial infection is part of the underlying disease or a consequence of critical illness, antibiotics will have an important role in the therapeutic plan. Choosing the best antibiotic regimen requires guidance from a bacterial culture and susceptibility test. This information will not be immediately available so the initial choice is typically made based on the most likely pathogen(s). Initial treatment with inappropriate or insufficient antimicrobial therapy increases both morbidity and mortality [1–3]. Antibiotic stewardship is the practice of minimizing bacterial resistance. The importance of responsible antibiotic stewardship in today’s ICU cannot be overemphasized. This responsibility necessitates that protocols and guidelines be established for the ICU facility and personnel regarding antibiotic selection, environmental surveillance, patient isolation, and monitoring culture results from the clinical laboratory. The potentially catastrophic impact that infection can have on patient survival requires early and effective intervention to optimize recovery. Appropriate patient diagnostic and monitoring procedures can provide insight into the status of the immune system and presence of likely pathogen(s). This information is vital to guide appropriate therapy and antibiotic selection. Currently, there is no routine laboratory test that will demonstrate specific problems with the immune response. Data collection begins with a thorough history and physical examination, followed by cage‐side point of care (POC) laboratory testing, clinicopathological laboratory testing, and diagnostic imaging. Careful assessment of the leukogram and each component of the white blood cell (WBC) line can provide a window into the status of the patient’s immune capabilities. Diligent monitoring is necessary to identify occult infections or failed antibiotic response. The history begins with the signalment (age, sex, breed) to identify any possible age, sex, or breed predispositions to immune disorders. Very young animals may have poor innate immunity due to failure of passive antibody transfer from the mother. Older animals are more likely to have renal or hepatic dysfunction leading to excessive or toxic drug concentrations at standard doses. Purebred animals may be at increased risk for genetic immune dysfunction, including IgA deficiency in Chinese Sharpeis, IgM deficiency in Doberman Pinschers, IgG deficiency in Weimaraners, and Chédiak–Higashi syndrome in Blue Smoke Persian cats. The patient’s past medical history can provide insight into concurrent disease states that might compromise the immune response (such as diabetes mellitus, hyperadrenocorticism) or medications that cause immunosuppression (glucocorticoids, chemotherapy drugs). Recurrent urinary tract or skin infections and chronic GI dysfunction may be important pathogen sources. A list of drugs that cause immunosuppression in the dog and cat is provided in Box 14.1. The history can also suggest likely exposure to pathogens, such as recent boarding, removal of ticks, vaccination history, exposure to endemic areas (such as leptospirosis or systemic fungi), or trauma. Known or suspected drug allergies (such as penicillin, cephalosporin or sulfa drugs) or intolerances (vomiting, diarrhea) should be noted and the offending agent(s) posted on the cage card and record. Past antibiotic treatment regimens should be recorded and evaluated when making the current antibiotic selection. Chronic history of recurrent infections (skin, urogenital, pulmonary) should be evaluated for indication of an inadequate immune response. Patient‐specific factors such as age, pregnancy, and preexisting conditions should all be discovered during anamnesis (history taking). These will have direct relevance when choosing appropriate antibiotic therapy. For example, it may be prudent to avoid certain drugs in animals with nervous system disorders. Imipenem can initiate seizures, aminoglycosides will worsen neuromuscular blockage in myesthenics, and beta‐lactams at high dosages can cause toxicity within the central nervous system (CNS). Pregnant animals have altered physiology, including larger plasma volume, increased renal blood flow, and hormonal influences on hepatic microsomal enzymes. There are no antibiotics approved for pregnant or lactating animals nor for most animals under 12 weeks of age. Blood flow to the placenta will limit fetal drug delivery, but ultimately the drug will pass across the placenta by passive diffusion. The physical examination begins with the temperature, pulse rate and intensity, respiratory rate and effort, capillary refill time (CRT), mucous membrane color, and skin turgor. Fever or hypothermia can be an indication of systemic inflammation. The pulse rate and intensity, CRT, and mucous membrane color are used to assess the perfusion status of the patient. Skin turgor, eye position in the orbits, and mucous membrane and corneal moisture are used to assess hydration. Pertinent physical examination findings suggestive of obvious or occult bacterial infection include purulent discharge; profuse and/or bloody diarrhea; heart murmur in a febrile patient; redness, pain, heat or swelling in any location; phlebitis or vasculitis; open contaminated wounds; and abdominal pain. The minimum laboratory database should include the packed cell volume (PCV), total protein (TP), blood glucose (BG), blood urea nitrogen (BUN), electrolytes, blood gas, coagulation profile, and urinalysis. A complete blood count (CBC) should be performed and a blood smear should be made to assess the WBCs (Box 14.2). The PCV and TP are evaluated for evidence of anemia, hypoproteinemia or severe dehydration. These can all result from systemic inflammation and may impact antiobiotic therapy. A microhematocrit tube buffy coat layer that is less than 1% or more than 3% may indicate an altered leukogram. Hypoglycemia may be present with sepsis. Hyperglycemia can be associated with a stress response or diabetes mellitus that can affect immune responses and predispose to infections. An elevation in BUN is concerning for concurrent renal disease which may affect the antibiotic selection and dose regimen. A low BUN brings concern for hepatic dysfunction and antibiotic metabolism. Electrolyte and acid–base status may influence antibiotic efficacy. Point of care ELISA tests may identify the presence of a specific pathogen. Diagnoses such as parvovirus, feline leukemia virus or feline immunodeficiency virus all have a negative impact on the immune response in an infected dog or cat. ELISA tests for Ehrlichia, Dirofilaria, Anaplasma, and borreliosis (Lyme disease) are available to identify sources of inflammation and guide treatment. The urinary tract can be a source of systemic bacterial entry. Identification of bacteria and WBCs on routine urinalysis may provide insight into a source of infection. The presence of casts could indicate renal tubular damage from antibiotic use (such as aminoglycosides) or worsening renal injury (see Chapter 13). Patients treated with aminoglycosides should have their urine sediment examined prior to administration of the drug each day. Blood is submitted for a CBC and serum biochemical profile. The biochemical profile is examined for evidence of renal or hepatic dysfunction. These are important in selection of antibiotics and dosing regimens. Dose adjustment may be appropriate in cases of renal disease for fluoroquinolones and other antibiotic metabolites that require renal excretion. Vancomycin is excreted by renal glomerular filtration while aminoglycosides are excreted by tubular diffusion, and cephalosporins and penicillins by tubular secretion. Metronidazole, macrolides, clindamycin, and tetracyclines require hepatic metabolism and dose adjustment may be appropriate in hepatic disease (see Chapter 18). Fluid or tissue samples are collected for cytology, specific antigen or polymerase chain reaction (PCR) testing, histopathology (when indicated), and culture and susceptibility testing. Blood can be tested for titers (antibody levels) for specific pathogens, such as Leptospira spp., rickettsial diseases, toxoplasmosis, and fungal diseases, although detectable levels may require convalescent titers performed at a later date. Table 14.1 lists sites and techniques for culture and sensitivity sample collection. Table 14.1 Sites and techniques for culture and susceptibility testing sample collection. Cytological examination of collected fluid or cells may allow identification of the infectious organism, and provide insight into the type of inflammation present. A gram stain is helpful in bacterial identification and to guide initial antibiotic selection. The presence of a large number of neutrophils (neutrophilic inflammation) is most commonly associated with bacterial infection or tissue necrosis. Mixed inflammation or pyogranulomatous inflammation (neutrophils and macrophages) is more commonly associated with fungal infection, foreign bodies, and mycobacterial infections. An abundance of eosinophils is often associated with allergic disease or parasites. The quantification of glucose in abdominal cavitary fluids is a useful diagnostic test. If the abdominal fluid glucose is >20 mg/dL lower than the patient’s peripheral blood glucose, a septic source is likely and surgical exploration of the abdomen is recommended [4]. Fluid collected directly from the abdomen or from a drain for analysis of glucose or lactate in postoperative patients does not provide the same diagnostic information [5,6]. Diagnostic data suggestive of bacterial infection include unexplained hypoglycemia; neutropenia or neutrophilia with left shift; degenerative or toxic neutrophils and bacteria seen on cytological examination of discharge, sputum, or sampled fluid; bacteriuria or pyuria; hyperbilirubinemia; hypoalbuminemia; moderate thrombocytopenia; and alterations in coagulation tests without obvious cause. Additional specific testing may demonstrate abnormalities compatible with the site of bacterial infection. These include valvular heart lesions on echocardiogram in febrile animal; free abdominal fluid or cavitary lesion(s) on radiographs or ultrasound; and purulent exudate on bronchoalveolar lavage or transtracheal wash. The criteria for diagnosing SIRS in the dog and cat are listed in Table 14.2. When present, these signs should prompt the clinician to intervene with early and appropriate antibiotic therapy as soon as possible and long before culture and sensitivity or bacterial titer results are available. Table 14.2 Criteria for the diagnosis of the systemic inflammatory response syndrome (SIRS) in the dog and cat. The patient must have two or more criteria. HR, heart rate; RR, respiratory rate; WBC, white blood cell. Evaluation of the immune status begins by interpreting the leukogram portion of the CBC results. This includes the total WBC count, complete differential, and WBC morphology. Leukocyte counts are relatively constant in health but will increase in response to infection, inflammation, tissue trauma or injury, stress, and immune stimulation. All leukocytes are derived from common hematopoietic stem cells in the bone marrow. The differentiation of progenitor cells into the various cells lines is signaled by various cytokines (Table 14.3). This process takes approximately six days – three days for proliferation and three days for maturation of the WBCs. Table 14.3 White blood cells and their contribution to immunity. The total WBC count on the leukogram is used to calculate the absolute numbers of WBCs found in the differential count. Normal leukogram reference ranges are typically 5.3–19.8 k/μL for dogs and 4–19 k/μL for cats and will vary by laboratory (Table 14.4). The total WBC count should consist primarily of PMNs and lymphocytes. However, presence of a leukocytosis does not automatically equate with infection. Inflammation, pain, stress, and trauma can all cause a stress leukogram. A stress leukogram is denoted by neutrophilia, lymphopenia, eosinopenia, and monocytosis. The most consistent of these findings is a lymphopenia. These changes occur due to the influence of catecholamines and/or glucocorticoids. The general patterns of the leukocyte response are shown in Table 14.5. Table 14.4 Laboratory reference ranges for canine and feline complete blood cell counts. fL, femtoliters; g/dL, grams per deciliter; HCT, hematocrit; HGB, hemoglobin; K/μL, thousand per microliter; M/μL, million per microliter; MCH, mean corpuscular hemoglobin; MCHC, mean corpuscular hemoglobin concentration; MCV, mean corpuscular volume; pg, picograms; RBC, red blood cell; WBC, white blood cell;/μL, microliter. Table 14.5 General patterns of leukocyte response. Source: Adapted from Rebar AH, MacWilliams PS, Feldman BF, et al. A Guide to Hematology in Dogs and Cats. Jackson Hole: Teton NewMedia, 2002 [33]. C, cats; D, dogs; Eos, eosinophils; Mono, monocytes; Seg, segmented neutrophils; WBC, white blood cells. A total WBC count can be estimated based on a cytology slide (Box 14.3) [7] or hand‐counted with a hemocytometer. When using an automated machine, be aware that other cells such as nucleated red blood cells and large platelets may alter total WBC counts and give inaccurate readings. Various diseases can interfere with normal leukocyte function. Glycosylation of granulocytes in chronic diabetes mellitus patients will decrease oxidative burst and phagocytic killing. Cushing’s patients may have a relative lymphopenia or decreased ligand affinity [8]. Hereditary diseases involving neutrophil function defects have been reported in Doberman Pinschers and Weimeraners [9,10]. Hereditary lysosomal storage diseases are reported in dogs and cats [11]. Leukocyte function testing is available only at research laboratories. These techniques allow specific testing of in vitro chemotaxis, oxidative burst, phagocytosis, killing, and activation marker expression in granulocytes. Lymphocytes are tested for proliferative response, B‐ or T‐cell receptor, and expression of other markers. Disorders of neutrophil function include canine leukocyte adhesion defect and cyclic hematopoiesis of Gray Collies [12,13]. Evaluation of a peripheral blood smear provides vital information regarding the cell populations and morphology (Figure 14.3). A systematic scan is required to obtain accurate and consistent results. The leukogram is interpreted in light of patient‐specific clinical information. The absolute cell counts are evaluated, not the percentages. Abnormal leukocyte morphology, presence of a left shift, monocytosis or persistent eosinophilia can each indicate an inflammatory response. Figure 14.3 Peripheral blood cells. (a) Normal canine neutrophil. (b) Feline band neutrophil. (c) Toxic canine neutrophil. (d) Normal canine small lymphocyte. (e) Reactive canine lymphocyte. (f) Neoplastic canine lymphocyte. Aqueous Romanowsky stain. Scale bar = 10 microns. Source: courtesy of Dr Robin Allison. There are several pathogens that may be diagnosed from a peripheral blood smear (Figure 14.4). Specific inclusion bodies in neutrophils and red blood cells (RBCs) can be found in canine distemper virus infections. Anaplasma phagocytophilum morulae can be found in canine neutrophils while Hepatozoon americanum gamonts are found in canine monocytes. Intracellular organisms may be identified in circulating granulocytes during bacterial or fungal infections. A review of cell morphology by a pathologist can provide valuable information and should be requested when there is a high index of suspicion for WBC abnormalities. Figure 14.4 Infected peripheral blood cells. (a) Circulating C. felis piroplasms in feline red blood cells. (b) Circulating large Babesia piroplasms in canine red blood cells. (c) Bacterial rods in a neutrophil from a septic cat. (d) Histoplasma capsulatum yeasts in neutrophil from a dog with disseminated histoplasmosis. Aqueous Romanowsky stain. Scale bar = 10 microns. Source: courtesy of Dr Robin Allison. Automated blood cell counters can often provide more consistent results and decreased inherent errors by approximately 5% [14]. However, the clumping of leukocytes or breakage of fragile cells can result in inaccurate counts. An automated hemocytometer may also incorrectly classify abnormal cells such as lymphoblasts and nucleated red blood cells. A WBC estimate on a stained blood smear should be compared to the automated count to identify cell or cell fragments that might change the count. Performing a manual differential as a POC test allows severe neutropenia or abnormal cell populations to be identified and addressed early. As the patient improves, the leukogram should show resolution of abnormalities such as a left shift and toxic changes. Recovery of lymphopenia or eosinopenia may precede clinical recovery. The basic characteristics of the individual WBC lines and their role in the immune response are listed in Table 14.3. Common causes of high and low cell counts in specific WBC cell lines in the dog and cat are listed in Table 14.6. A bone marrow aspirate or core biopsy is indicated when there is a persistent moderate‐to‐severe neutropenia without a left shift or toxic change, particularly when there is no evidence of a septic source; pancytopenia with nonregenerative anemia; monocytosis with depressed numbers in other cell lines; myelophthisis; myeloid or lymphoid leukemia; and subleukemic or aleukemic leukemia. Table 14.6 Common causes of high and low white blood cell line numbers in dogs and cats. The normal immune response to an antigen or pathogen should increase the number and enhance the function of neutrophils at sites of injury and inflammation. Neutrophils (or PMNs) exist in the body in two pools of approximately equal size: circulating and marginated. Cytokine release will stimulate neutrophil release from the bone marrow. Marginated neutrophils roll along the endothelial surfaces of capillaries and interact with low‐affinity selectin. Firm adhesion occurs through integrins, with the PMN ultimately migrating through the capillary toward sites of infection or inflammation by chemotaxis. The immune stimulation of neutrophils by cytokines enhances antimicrobial cell function and phagocytic killing. The presence of lipopolysaccharides from gram‐negative bacteria will also increase neutrophil oxidative burst and adhesion to the endothelium, but will decrease the ability of neutrophils to marginate across vascular endothelium. The presence of a neutrophilia can imply infection, inflammation, or a stress response due to endogenous or exogenous corticosteroids. An extreme neutrophilic leukocytosis (leukemoid response) or a marked lymphocytosis requires further diagnostic testing to rule out granulocytic leukemia, lymphocytic leukemia, and lymphoma. An abnormally low number of neutrophils may be due to sequestration or consumption due to acute overwhelming inflammation or infection, peripheral destruction or decreased central marrow production. Neutropenia in critically ill patients suggests dysfunction or incompetence of the immune response. Neutropenia with a left shift further indicates that the inflammation is acute or overwhelming and that the bone marrow has not yet responded or cannot keep up with demand for neutrophil response to tissue damage or infection. A left shift is identified if immature or band neutrophils make up greater than 3% of the total neutrophil population. Band neutrophils have a horseshoe‐shaped nucleus with smooth, parallel sides that lack the typical segmental constrictions seen in nuclei of mature neutrophils. A left shift may be further characterized as “degenerative” if the number of band neutrophils is greater than 10% of the total neutrophil count, the total segmental neutrophil count is within or below the reference interval, or the total number of bands (with metamyelocytes and myelocytes included) is greater than or equal to the total number of segmented neutrophils [14,15]. The presence of a degenerative left shift indicates that the tissue demand for WBCs is surpassing the rate of bone marrow production and is associated with a poor prognosis. Evaluation of neutrophil morphology is important for accurate interpretation of the leukogram and neutrophil count and may also provide prognostic information. Abnormal neutrophil morphology can represent increased bone marrow recruitment or band release, accelerated neutrophil development or aging. Certain morphological changes (inclusions) are pathognomonic for intracellular infection or particular infectious etiologies. Toxic change in neutrophils is identified by cytoplasmic basophilia with vacuoles. Circulating toxins, infection or accelerated neutrophil production by the bone marrow can cause these morphology changes. The altered cytoplasmic appearance is due to the presence of remnants of organelles and ribosomes from early cellular development. Giant neutrophils, aberrant nuclear shapes (such as ring forms), and Dohle bodies also indicate toxicity. Dohle bodies are leftover precipitates of rough endoplasmic reticulum that appear as 1–3 µm light blue‐gray oval inclusions near the periphery of the neutrophil. They are frequently seen in the neutrophils of healthy cats, so the presence of Dohle bodies in canine neutrophils is of much greater significance. Neutrophil hypersegmentation may result from sample aging or drugs such as corticosteroids. Several hereditary diseases can affect neutrophil morphology. In Chédiak–Higashi syndrome of Blue Smoke Persian cats, the neutrophils and other nucleated cells often contain large peroxidase‐positive cytoplasmic granules filled with lysosomal enzymes [16]. Lysosomal storage disorders are characterized by a lack of mobilization of the lysosomal granule contents, resulting in PMN dysfunction and an increased susceptibility to infections. In the neutrophil anomaly of Birman cats, reddish‐purple cytoplasmic granules can be seen within the neutrophils [17]. Pelger–Hüet anomaly seen in both dogs and cats is characterized by hyposegmented nuclei with coarse, clumped chromatin [18,19]. Because the cells appear hyposegmented, they may be inadvertently classified as band neutrophils and misinterpreted as a severe left shift consistent with inflammation. Lymphocytosis, while infrequent in critically ill patients, can be seen with chronic antigenic stimulation, recent vaccination, or neoplasia. A transient epinephrine‐induced lymphocytosis is common in fractious cats. Critically ill dogs and cats should have a stress‐induced lymphopenia, making the presence of lymphocytosis cause to test for hypoadrenocorticism (Addison’s disease) or relative adrenal insufficiency. A leukocytosis with a marked lymphocytosis could be indicative of lymphoid leukemia and warrants evaluation of lymphocyte morphology. The neoplastic lymphocytes appear larger than normal cells and have increased basophilia to the cytoplasm, large round nuclei, coarse granular chromatin, and nucleolar whorls. Due to abnormal size and morphology changes, these cells may not be accurately recognized or classified as lymphocytes by in‐house analyzers, requiring examination of a blood film. Occasionally patients with leukemia will have marked increases in normal‐appearing small lymphocytes. Special stains can be used to help differentiate acute myelogenous leukemia from lymphoblastic leukemia. A concurrent nonregenerative anemia is common due to bone marrow crowding of red blood cell precursors. The granulocyte and platelet lines may also be decreased. Lymphopenia (750–1500 k/μL) is the most reliable and consistent clue to making the diagnosis of a stress leukogram. Unfortunately, this is indistinguishable from lymphopenia of acute viral or bacterial infection. Marked lymphopenia warrants consideration of other differential causes such as lymphoma, chylous effusion, and lymphedema. Declining lymphocyte counts in an apparently healthy patient may indicate impending illness but cannot be distinguished from a corticosteroid effect. Persistent lymphopenia can suggest ongoing disease. Lymphocyte morphology should be assessed on a blood smear. Reactive lymphocytes are larger in size, have increased basophilic cytoplasm, and have larger nuclei with finely granular chromatin. The presence of reactive lymphocytes indicates immune system reactivity. Large granular lymphocytes may represent the presence of circulating natural killer, cytotoxic T‐lymphocytes, or neoplastic lymphoblasts. Diseases of abnormal lymphocyte function include severe combined immunodeficiency and other rare congenital disorders [20–22]. These patients may have normal lymphocyte numbers with abnormal function. While a mild increase in monocytes can accompany normal endogenous stress response, a more marked monocytosis is suggestive of inflammation. Monocytosis is expected in diseases with high phagocytic activity, including immune‐mediated hemolytic anemia, tissue necrosis, foreign body reactions, and organisms associated with pyogranulomatous or granulomatous inflammation. These organisms include fungi, filamentous bacteria (Nocardia, Actinomyces, Dermatophilus), mycobacteria (tuberculosis, leprosy, atypical mycobacteriums), Pythium, Toxoplasma, and others. When evaluating a peripheral blood smear or cytology sample, it is important to check the monocytes carefully for inclusions or intracellular organisms. When a monocytosis is seen concurrently with other WBC line deficiencies, several differential diagnoses, including erythrophagocytosis, hemophagocytic syndrome, and monocytic neoplasia, should be considered. Monocytes are of low frequency in the bone marrow and circulating blood and are the major source of colony stimulating factors and cytokines. They are less effective than neutrophils at microbial defense. Once they enter the tissues, monocytes transform into macrophages that contain more granules and proteolytic enzymes. Their function is to digest foreign material and dead cells. Macrophages are important for antigen processing and presentation to T‐lymphocytes. These tissue macrophages may survive for prolonged periods. Monocyte‐derived macrophage populations include the macrophages and histiocytes found in exudates, pleural and peritoneal macrophages, pulmonary alveolar macrophages, connective tissue histiocytes, and also the Kupffer cells of the liver. The macrophages of the spleen, lymph nodes, and bone marrow are collectively referred to as the reticuloendothelial system. There are several organisms that can survive and replicate within macrophages including Mycobacteria spp., Rickettsia spp., Leishmania spp., and Toxoplasma spp. Like monocytes, eosinophils are typically of low frequency in the bone marrow and circulating blood. These are primarily tissue‐dwelling cells and can accumulate in tissues without a circulating eosinophilia. Eosinophilias may be seen in allergic reactions, parasitic infections (GI, heartworm, larval migrans), and inflammation (feline asthma, allergic gastroenteritis). A persistent eosinophilia is typically associated with an ongoing systemic hypersensitivity. Patients with hypoadrenocorticism often have a persistent eosinophilia and lack a mature neutrophila. Other less common causes of eosinophila include neoplastic and paraneoplastic conditions, hypoadrenocorticism, systemic mastocytosis, and idiopathic hypereosinophilic syndrome. Basophils also exist in small numbers in the bone marrow and circulating blood. Basophilia can be seen in similar conditions as eosinophilia. Choosing the appropriate sample, collection technique, and timing is important for obtaining the most accurate bacterial culture and susceptibility information. Common sample sites and techniques for sample collection are provided in Table 14.1. If a “dirty” site is to be sampled, the area should be aseptically prepared and sterile technique used for sample collection. Any exudate or necrotic debris should be cleared away prior to sample collection. While it is ideal to collect samples for culture prior to treating, rational antimicrobial therapy should not be delayed in order for samples to be collected [1]. Appropriate broad‐spectrum antimicrobial therapy should be instituted as soon as possible to avoid increased mortality [2]. Radiographs of the chest and abdomen and abdominal ultrasound may help to identify sources of infection. Particular attention is given to the genitourinary tract, GI tract, and respiratory tract since these nonsterile sites provide common sources of bacterial colonization. The presence of free air in the closed abdomen is indicative of an anaerobic pathogen or rupture of a hollow viscus and either scenario necessitates exploratory surgery of the abdomen. Echocardiogram will identify valvular lesions compatible with bacterial endocarditis and can help identify cardiac systolic dysfunction consistent with SIRS. Focused ultrasound of the thorax may be helpful for pleural space disease and sample collection. Free fluid or loculated fluid pockets should be tapped and the fluid evaluated. Advanced imaging, such as computed tomography (CT) and nuclear magnetic resonance imaging (nMRI), may be used to better define pathology in bone and in the soft tissue structures of the CNS and other difficult‐to‐assess areas of the body. The Rule of 20 provides an important checklist of monitoring priorities for the patient with infection or immune compromise. A single leukogram provides the clinician with a snapshot of the immune status of the patient at one moment in time, but does not provide information about chronicity or trend of change. Serial assessment of the leukogram (every 48–72 hours) is needed to determine improvement or deterioration of the WBC response to immune stimulation. Physical parameters (such as body temperature, body weight, peripheral perfusion parameters, hydration status, and mentation) and POC minimum database parameters are monitored several times daily to assess response to therapy and identify new clinical problems. The discovery of a new fever or infection or a failed antibiotic response necessitates a careful review of the antibiotic and environmental protocols established by the antimicrobial stewardship program of the facility. Antimicrobial Stewardship is the practice of minimizing the emergence of antimicrobial resistance. Antibiotics are used only when there is evidence from a positive culture result or a high index of suspicion (Table 14.7) that bacterial infection is an important component of the disease process. Table 14.7 Patient and laboratory factors commonly associated with bacterial infection. The presence of two or more of these factors supports the presence of a bacterial pathogen as a component of the underlying disease process. The three Ds are three simple steps to follow: decontaminate, design, and deescalate [23]. Decontaminate is to reduce bacterial exposure, particularly to resistant bacteria. This includes treating patients in order of least risk to most risk and appropriate early discharge of patients from the hospital to minimize exposure. Removing resistant microbes from the environment is paramount. Proper hand washing, barrier nursing techniques (such as gloves, gowns; see Box 20.9), and use of bandages for wounds are important to prevent horizontal transmission. Design means to select the most appropriate drug, drug dose, and dosing interval for the infection. This will require identification of the site or body system and the most likely bacterial pathogen(s) involved (Table 14.8). It is important to select appropriate empirical antibiotics before culture results are available. The decision to use antibiotics is made based on information obtained from history, physical examination, laboratory data, imaging, and sample analysis. Table 14.8 Common bacterial pathogens associated with various organ systems in the dog and cat. Finally, deescalate starts with not using antimicrobials when alternate therapy is equally effective. Other components of deescalation include decreasing hospital length of stay, length of antimicrobial therapy, and decreasing the use of invasive devices (such as catheters) whenever possible. When culture results are available, antibiotic therapy should be deescalated to the most appropriate narrow‐spectrum or first‐line antibiotic(s). Individual hospital policies providing guidelines for the use of antimicrobial agents should be designed to minimize development of multiresistant bacteria. Patients infected with resistant bacteria are likely to experience ineffective treatment, recurrent infection, delayed recovery, or even death. Antibiotic use to prevent infection is called chemoprophylaxis, and has been employed in humans to: The risks of infection must be weighed against the potential side‐effects of the drug and the development of resistant bacteria. The institution of antibiotic prophylaxis in the first situation is based on the likelihood that the exposed animal will be infected by the known pathogen. It is ideal to prevent infection by utilizing aseptic technique during procedures rather than administering chemoprophylaxis. The goal of antimicrobial therapy is to assist the patient in containing and eliminating infection without causing toxicity, adverse side‐effects or drug interactions, or increasing antimicrobial resistance. Factors to consider when selecting the “best” initial antibiotic regimen include infection location and likely pathogens, patient (host) status, other drugs being administered, and specific characteristics of the selected drug. A schematic of drug and patient factors to consider during antimicrobial selection is shown in Figure 14.2 [24]. Reviewing the list of common bacterial pathogens in the dog and cat (see Table 14.8), as well as the antibiogram (see Figure 14.5) specific for the ICU facility, will provide insight into which bacteria to target with a particular antibiotic. Figure 14.5 Antibiogram. This is one example format for charting bacterial isolates and their antibiotic susceptibilities. Results from each culture submitted from ICU patients in a specific facility should be entered into the antibiogram. The antibiotics available and tested are listed on the horizontal upper axis and the bacteria species isolated and tested are listed along the vertical axis. There are several options for recording results. If there are few results available, put an S, R or I in the appropriate square to indicate whether that bacteria was sensitive, resistant or had an intermediate response to the antibiotics tested. If there were multiple culture results for the one bacterial species, the % of isolates sensitive to the antibiotics can be noted under the antibiotic column, with the number of isolates noted by the bacterial identification. Common parenteral antibiotics used to treat critically ill dogs and cats are listed in Table 14.9, along with the spectrum, mechanism of action, dosage, and a few precautions for each category of agent. It is important to review the drug insert prior to prescribing any antibiotic. For companion animals, most antibiotic use is extra‐label and subject only to scientific justification. The cost of ongoing therapy can also be a factor when selecting antimicrobial agents, particularly in patients who will require prolonged therapy. Table 14.9 Antibiotics commonly used in the veterinary ICU, arranged by class. Side‐effects, mechanism of action, and spectrum of activity are noted [24,43,44]. (c) cat; (d) dog; GI, gastrointestinal; PAE, postantibiotic effect. The characteristics of specific antibiotics(s) must be considered when making an initial empiric selection. Is the antibiotic bactericidal or bacteriostatic? What is its spectrum of activity against gram‐positive, gram‐negative, aerobic, anaerobic or facultative bacteria? Will the drug reach appropriate concentrations in the target tissue? What will be the route, frequency, and cost of administration? All of these as well as any potential adverse effects or drug interactions are important to consider (see Figure 14.2). The concentration of the antimicrobial drug in the targeted tissues will depend on the drug solubility, ionization, protein binding, and pKa. Additional drug characteristics to consider when prescribing a drug and dosing regimen for the ICU small animal patient are presented in Chapter 18. Antimicrobial penetration into barrier‐protected sites such as the eye, prostate, and brain requires selection of drugs that are lipid soluble, nonprotein bound, and transported by a transcellular route. Antibiotics such as chloramphenicol and trimethoprim‐sulfa can penetrate these barriers. If there is inflammation of the blood–brain, blood–aqueous, or blood–prostate barriers, then additional drugs may penetrate. Liposome‐entrapped antimicrobial drugs have enhanced activity against facultative intracellular pathogens, and in some cases (amphotericin B) have decreased systemic toxicity. In general, bactericidal antimicrobials cause interference of bacterial cell function that is lethal to the bacteria (in vitro). These drugs can target the cell wall biosynthesis (beta‐lactams, polyene antifungals), cause cell membrane leakage (polymyxin), damage DNA (fluoroquinolones) or damage multiple bacterial ribosomal subunits (macrolides: erythromycin, azithromycin). The major classes of bactericidal antibiotics, regardless of drug–target interaction, stimulate the production of highly deleterious hydroxyl radicals. This occurs in both gram‐positive and gram‐negative bacteria, and ultimately contributes to cell death [25]. Bactericidal antibiotics are preferred for life‐threatening infections when host defense mechanisms or vital organs are at risk and when patients are immunosuppressed. Bacteriostatic drugs inhibit cell growth by damaging a single ribosomal subunit (tetracyclines) or disrupting a metabolic pathway (sulfonamides). Bacteriostatic drugs have not been shown to produce hydroxyl radicals. The action of the antibiotic, however, will ultimately be dependent on the concentration of the drug in the target tissue. Bactericidal and bacteriostatic antibiotics should not be combined because the bacteriostatic drug may neutralize effects of the cidal drug. However, combination of two bacteriostatic drugs that act synergistically can result in a bactericidal effect. Culture and susceptibility (also called sensitivity) results will be used to determine which specific antibiotic is more likely to be effective in treating the infection in vivo. Several different parameters may be reported. The minimum inhibitory concentration (MIC) is a measure of the lowest drug concentration inhibiting bacterial growth (105 cfu/mL). Should the amount of drug required to inhibit growth exceed what can be safely administered, the bacterium is labeled as “resistant” to that drug. These measures are often based on tissue levels, but in some tissues or fluid (example, urine), the concentration of the antibiotic may be much higher, resulting in clinically therapeutic levels. The minimum bactericidal concentration (MBC) measures bactericidal activity of the antibiotic. Unfortunately, bactericidal and bacteriostatic activity are determined in vitro and do not always translate to in vivo situations. It may be difficult to safely achieve a bactericidal concentration of a drug at the site of infection in a particular patient. If the MBC is not achieved at the site of infection, even a bactericidal drug can be bacteriostatic in vivo. More recently, the mutant prevention concentration (MPC) has been described as a novel measurement of in vitro susceptibility or resistance and is based on the testing of larger bacterial inocula (>/= 109 cfu/mL) [26]. The MPC defines the lowest drug concentration required to block the growth of the least susceptible cell present in high‐density bacterial populations. MPC testing is assessed on microorganisms considered susceptible to the drug by MIC testing. Doses that achieve a concentration that is higher than the MIC but lower than the MPC are described as falling within the mutant selection window (MSW). This is undesirable because it will encourage the emergence of resistant strains (mutants). Failure to achieve the MPC in vivo may allow emergence of resistant microbes, especially in at‐risk or immunosuppressed patients, ultimately leading to treatment failure. The range of bacteria affected by the antibiotic is termed the spectrum and describes the effect of the antibiotic against gram‐positive (Gram +) or gram‐negative (Gram −) bacteria. Antibiotics that kill or inhibit a wide range of Gram + and Gram − bacteria are said to be broad spectrum. If effective mainly against Gram + or Gram − bacteria, they are narrow spectrum. If effective against a single organism or disease, they are referred to as limited spectrum. Bacteria can be classified into aerobes and anaerobes. Aerobic bacteria require oxygen to remain alive, while anaerobic bacteria do not rely on oxygen for survival and may die in its presence. This anaerobic type of bacteria does have a growth advantage in areas of the body unexposed to oxygen, and may be a source of infection associated with leakage or absorption of GI bacteria, deep wounds, open fractures, and surgery (abdominal or implant orthopedics). Facultative bacteria can make adenosine triphosphate (ATP) by aerobic respiration if oxygen is present, but are capable of switching to fermentation or anaerobic respiration if oxygen is absent. Mixed infections involve complex interactions between facultative bacteria and strict anaerobes, many of which possess intrinsic pathogenicity. The best therapeutic results are realized with antimicrobial drugs that are active against both types of microorganisms. This emphasizes the importance of culture and susceptibility testing. For example, the facultative bacterium Escherichia coli is often responsible for acute peritonitis and sepsis associated with bowel perforation. However, anaerobes, particularly Bacteroides fragilis, play the seminal role in subsequent abscess formation. Treatment of only the facultative bacteria, without adequate antibiotic coverage for anaerobic bacteria, leads to clinical failures with complications of abscess formation. Such therapeutic misadventures have been witnessed in the treatment of mixed infections with cephalosporins and penicillins that lack significant activity against anaerobes. Similarly, use of metronidazole or clindamycin as a single agent is associated with failures caused by infection with facultative bacteria. Antimicrobial drug combinations are often indicated in critical patients with compromised defenses or immune impairment. Infections such as peritonitis, pyothorax, aspiration pneumonia, and urogenital infections often have mixed bacterial populations. Combining drugs that have different mechanisms of action can widen the target for bacterial destruction and reduce the likelihood of microbial resistance during treatment. In some cases, combining two agents can decrease dose‐related toxicity (flucytosine + amphotericin B). Keep in mind that not all drugs work synergistically and not all drug combinations are desired. Some combinations can have added or synergistic toxicity. Combinations that destroy normal GI flora may promote superinfection, a second infection superimposed upon the first. Synergistic antibiotic combinations used in dogs and cats are listed in Table 14.10. Table 14.10 Clinically useful antimicrobial drug combinations. Source: Adapted from Giguère S, Prescott JF, Dowling PM. Principles of antimicrobial drug selection and use. In: Antimicrobial Therapy in Veterinary Medicine, 5th edn. Ames: John Wiley & Sons, 2013 [1]. The most appropriate route of administration for antimicrobial agents in the ICU is by IV injection, providing 100% bioavailability of the drug. Factors such as poor perfusion, dehydration, overhydration, and abnormal GI function can affect the antibiotic plasma drug concentration (PDC) after subcutaneous, intramuscular or oral administration (see Table 18.1, Chapter 18). Administration of a loading dose by IV injection, as directed by drug insert, can be used to rapidly achieve therapeutic PDC. Constant rate infusions may be indicated to maintain therapeutic drug levels throughout the dosing interval for antimicrobials, requiring frequent administration to maintain PDC above MIC (also called time‐dependent antibiotics). Suboptimal antibiotic concentrations increase the likelihood of treatment failures, antibiotic resistance, and patient toxicity. As patients recover and transition to step‐down wards or discharge to home care, they will be transitioned to oral medications. Adequate GI function must be achieved prior to this transition. Whenever possible, drugs with a large therapeutic index should be chosen. This means there is a large window between the amount of drug that causes therapeutic effect and the amount of drug that causes toxicity. Knowledge of possible adverse drug reactions for the selected agent(s) should be reviewed and orders established for monitoring and intervention, should it become necessary. Idiosyncratic reactions are independent of dose and duration and cannot be predicted. Critically ill dogs and cats are typically on multiple medications including IV fluids, antimicrobials, and nutrition. It is important to consider how these may interact with one another both before and after administration. Tetracyclines mixed with calcium‐containing fluids and enrofloxacin combined with magnesium‐containing fluids will chelate, thus reducing drug delivery. Cephalosporins and aminoglycosides provide a synergistic antibacterial combination in vivo, but will precipitate when mixed in solution together. Some drug interactions can be used to advantage. The drug probenecid has been used to block renal excretion of ampicillin, thereby increasing the PDC of ampicillin. Antibiotics, antifungals, and other drugs can alter hepatic microsomal systems. This may put patients at risk for hepatic damage, or may alter drug metabolism and excretion. The administration of antacids, corticosteroids, immunosuppressants, and p450 enzyme inhibitors or promoters will also alter drug efficacy. Corticosteroids can delay healing, reduce fever, suppress inflammation, impair phagocytosis, and impair the normal immune response. Concurrent administration of corticosteroids with antimicrobials, while poorly investigated in companion animals, is typically not recommended. Exceptions to this rule exist. Patients that have immune‐mediated disease and a bacterial infection will require antibiotic and immunosuppressive therapy. In addition, when the inflammatory response to the bacterial infection is potentially life threatening (such as bacterial meningitis or fungal pneumonitis), the combination of corticosteroids and antibiotics is often recommended. The administration of short‐term high doses of corticosteroids has been shown to be harmful in humans and animals with septic shock [27]. The administration of long‐term low‐dose corticosteroid therapy (with hydrocortisone) has shown some promise in human patients but there is no evidence to support this in companion animals at this time [28]. Some antibiotics will have a postantibiotic effect (PAE), meaning they continue to act after the drug has been removed. Proposed mechanisms by which the PAE occurs include nonlethal damage induced by the antimicrobial agent and a limited persistence of the antimicrobial agent at the bacterial binding site [29]. The specific microorganism‐antimicrobial combination is the most important factor to influence the presence and duration of the PAE. Additional factors include the antimicrobial concentration and the length of antimicrobial exposure. Most antimicrobial agents produce a PAE when tested against Gram + cocci. However, against Gram − bacilli, beta‐lactam antibiotics (except for imipenem) have a minimal, or even a negative, PAE. Aminoglycosides, inhibitors of protein and nucleic acid synthesis, and fluoroquinolones have PAEs against Gram − bacteria that range from one to four hours. Extending the dosing interval of an antimicrobial agent (such as an aminoglycoside) that produces a PAE has several possible advantages, most notably in decreasing undesirable effects of the drug. The concern for surgical bacterial contamination during “clean” surgical procedures (such as clean, clean with prosthesis, and clean with contamination) has lead to the use of perioperative antibiotics in surgical procedures. Short‐term therapeutic doses of antibiotics appropriate for the potential pathogens have been recommended for clean procedures, such as intraabdominal surgery or surgical insertion of implants. The antibiotics need to be in the tissues at the time of the surgery and potential contamination, requiring antibiotic administration before and often during surgery. There is no apparent benefit of antibiotic administration in these patients after the surgical wound has been closed, with continued antibiotic administration often causing resistance among bacteria. A typical perioperative antibiotic regimen would incorporate the IV administration of either a first‐generation cephalosporin or beta‐lactam antibiotic at the time of patient induction. The antibiotic dose is repeated every 1–2 hours during the surgical procedure. Clean or clean contaminated wounds are given 1–2 doses of antibiotic postoperatively. Adequate lavage will minimize bacterial colonization. Submission of tissue cultures will rapidly identify persistent flora. Contaminated or dirty surgical procedures will have an existing established infection at the time of surgery and should already be receiving antibiotic therapy prior to and during surgical intervention. Restricting the use of certain antibiotics or classes of antibiotics has been shown in human ICUs to reduce the appearance of nosocomial and resistant bacterial populations [30]. Additional advantages include decreased pharmacy expense and avoidance of adverse drug reactions. It is important to create an antibiotic protocol for the ICU and ensure that personnel adhere to the guidelines of antibiotic selection, utilization, and environmental bacterial surveillance. Available antibiotics and their action, spectrum, potential toxicity, and other specific notes are presented in Table 14.9. First‐line antibiotics include ampicillin and amoxicillin with or without potentiating agents; first‐generation cephalosporins; trimethoprim‐sulfonamide combinations; doxycycline; and metronidazole. When co‐infection with Gram − aerobes and/or anaerobes is suspected, additional antibiotics should be combined with first‐line agents until culture results are available. Appropriate choices may include amikacin, gentamicin, clindamycin, and metronidazole. For aggressive anaerobic coverage, metronidazole is given IV. The addition of an aminoglycoside is strongly recommended for serious infection from Gram − organisms. Third generation cephalosporins can be used if renal function is compromised. Appropriate doses and dosing intervals for all agents is emphasized. There is some evidence in human ICUs that combination therapy with narrow‐spectrum agents over prolonged periods may help curb resistance to broad‐spectrum antibiotics while still providing effective treatment of serious infections. Treatment with potent, broad spectrum drugs (imipenem, meropenem, third‐generation cephalosporins) should be reserved for life threatening infections. Therapy should be deescalated as soon as possible. The culture and sensitivity will direct this final antibiotic choice, with the safest, most effective narrow‐spectrum drug chosen whenever possible. Active charting of bacterial cultures and susceptibilities will create an antibiogram. This is used to identify emerging resistance to first‐line antibiotics and assist in making any changes in the hospital antibiotic protocol deemed necessary. It is also examined for an indication of probable nosocomial pathogens in hospitalized patients. An example of a format for an antibiogram is presented in Figure 14.5. Though all cultures are tracked, the bacteria that most commonly develop resistance (Klebsiella pneumoniae, Enterobacter spp., Pseudomonas aeruginosa and Staphylococcus spp.) are closely monitored for evidence of resistance to the first‐line antibiotics recommended by the hospital protocol. The frequency of charting may depend on the patient population of the facility, with higher populations warranting more frequent charting (such as 6–12‐month intervals). When resistance is found, the origin of the infection and the antibiotic prescribed should be investigated. A pattern of resistance found in infections treated recently at a veterinary facility or bacterial isolates atypical of naturally occurring infections in small animals (such as Morexella spp., Acinetobacter spp., Serratia spp., Enterococcus spp.) suggest that the infection may be nosocomial. The first animal that presented to the hospital with the identified resistant infection most likely did not develop the resistance to that first‐line antibiotic within your hospital environment. Persistent resistance patterns for previously susceptible bacteria suggest that resistance has occurred to the first‐line antibiotics in your hospital. The hospital antibiotic protocol should be amended to direct prescribing of a different first‐line agent or agents. Carbapenems, third‐generation cephalosporins, and fluoroquinolones are avoided as first‐line agents due to the high incidence and rapid onset of resistance. Potent antimicrobial agents such as vancomycin, tigecycline, and imipenem should be reserved for resistant infections, with their use guided by culture and susceptibility testing. The reservation of life‐saving antimicrobial agents (vancomycin, tigecycline) for use in resistant human infections is an ongoing ethical debate. In veterinary medicine, we are fortunate in that we do not routinely face infections with this degree of antimicrobial resistance. Only continued ethical and appropriate antimicrobial stewardship will prevent escalation of this problem. Antibiotic rotation is the strategy used in human ICUs where a class of antibiotic or a specific antibiotic is withdrawn from use for a defined period of time and reintroduced at a later point in an attempt to limit bacterial resistance. There is limited clinical data available as to its effectiveness, with the rotation reserved for facilities with a high incidence of resistance or nosocomial infections. Limited antibiotic rotation has been employed at some veterinary facilities with success. Nosocomial infections are defined as infections acquired from the hospital environment that develop more than 48 hours after admission to hospital. These infections are generally more serious and difficult to treat because the patient is weaker and less able to combat an infection. The bacteria are likely antibiotic resistant and more virulent. Conditions in busy veterinary facilities are conducive to the development of nosocomial infections and include patient crowding, frequent broad‐spectrum antibiotic usage, inappropriate use of barrier techniques, and inadequate hand hygiene. Sources of patient contamination are plentiful, including cages, work surfaces, examination tables, sinks, floor drains, and equipment (clippers, pulse oximeter probes, blood pressure cuffs, endotracheal tubes, etc.). Identification, treatment, and containment of nosocomial bacterial infections are challenging and expensive. A complicated infection is a bacterial or fungal infection associated with a structural or functional abnormality. These abnormalities may be congenital (e.g. patent urachus), functional (e.g. dialysis dependent), or iatrogenic (e.g. orthopedic implant). Animals in the ICU may have multiple catheters (such as intravenous, urinary, central lines), tubes (such as chest tubes, feeding tubes, oxygen cannulas), and life‐saving invasive procedures (such as peritoneal dialysis, mechanical ventilation, surgical cutdown) that predispose them to complicated infections. The causative bacteria are often antibiotic resistant, residing in fibrin tags or biofilms formed around the tips of the catheters or tubing. While appropriate antibiotic therapy is vital to a successful outcome, the indwelling device must be removed to achieve effective decontamination. Bacterial resistance is acquired when antibiotic‐resistant bacterial strains develop from formerly antibiotic‐susceptible bacteria. This can result from a mutant gene or altered DNA sequence. Plasmids are small molecules of altered DNA that can be transferred between bacteria for the rapid spread of antibiotic resistance among bacterial populations. Stimuli for the development of bacterial resistance include repeated antibiotic exposure, widespread antibiotic use (such as with fluoroquinolones), incorrect antibiotic dosing, or insufficient duration of antibiotic therapy. The importance of bacterial resistance has been demonstrated in human medicine, with the development of methicillin‐resistant and vancomycin‐resistant Staphylococcus aureus, enterococci, and Gram − bacteria. Isolation of methicillin‐resistant Staphylococcus aureus from small animals has been reported and causes similar therapeutic concerns for the veterinarian and facility as experienced in human medicine. Each hospital should have a structured antibiotic stewardship program, to include hospital hygiene, barrier nursing techniques, staff educational programs, patient isolation area, and a strict antibiotic policy for restricting and guiding antibiotic use. Hand washing is the single most important way to reduce microorganism transfer and hospital‐acquired infection. Hands can be washed with soap and water, or alcohol‐based hand rinses (70% ethanol). Noncompliance with hand‐washing protocols is the greatest obstacle to reducing pathogen transfer from patient to patient. Other barrier nursing techniques are discussed in Chapter 20. Exam gloves are primarily to keep the staff’s hands clean and do not provide an adequate barrier to prevent transmission of bacteria or viruses between animals. The use of gloves does not replace hand washing. Strict aseptic techniques must be applied when inserting indwelling catheters, performing venipuncture, aspirating cells or fluids, or performing invasive procedures such as mechanical ventilation, bone marrow sampling, or peritoneal dialysis. Surgical suites, ward and ICU cages, scrub sinks, tables, and treatment surfaces should have a regular routine of cleaning with bactericidal cleansers. Typically, the most appropriate products are quaternary ammonium compounds or accelerated hydrogen peroxide cleaners. Drains in the wards and runs must be cleaned and sanitized on a routine basis. Items such as hair clippers, thermometers, stethoscopes, and otoscopes must be carefully cleaned and monitored for contamination. Mop buckets and cleaning solutions must be changed frequently. Routine cultures of the environment can be monitored in hospital areas where nosocomial infections may originate, such as common surfaces (surgical suites or prep tables, cage surfaces) and common equipment (such as surgical ventilator and radiology tables). However, careful hospital‐wide surveillance of the resistance patterns of cultured organisms will be more effective for identification of nosocomial and resistant infections. Patients should be placed into isolation when they have a contagious infectious disease, or when they are known or suspected to have a multiresistant pathogen (such as open surgical wounds). The list of possible pathogens or problems requiring patient isolation includes, but is not limited to, the following: Salmonella, Campylobacter, parvovirus, Giardia, Cryptosporidium, psittacosis, canine distemper virus, influenza, methicillin‐resistant Staphycoccus infections, plague, rabies, tularemia, infectious canine tracheobronchitis, infectious feline upper respiratory diseases, feline distemper, and resistant bacterial strains. Isolation units are separated from the general ICU or hospital population and should have separate ventilation and filtered air. Ideally, the personnel are dedicated to the area and do not work with the general ICU population during their shift. Alternately, staff may handle these patients at the end of their shift. Hand washing and gloves are mandatory, as are protective gowns, masks, caps, and booties. Footbaths may be appropriate to clean the shoes of personnel when entering and leaving the isolation facility. Patients should be minimally handled or moved within the isolation unit to reduce surface contamination. Whenever possible, diagnostic, surgical, and other procedures should be performed within the unit, avoiding common patient areas in the general hospital environment. When the procedure can only be performed in the main patient areas, precautions must be taken to avoid fecal, urine, or secretion contamination of the area, and proper cleaning and disinfection should be performed immediately afterward. All instruments and equipment used to monitor and treat isolation patients is dedicated to that area and should be thoroughly cleaned between patients. Disposable items should not be reused. Ideally, patients should have a separate entrance and a separate elimination area or be allowed to eliminate in their cages to avoid contamination of common areas. Surfaces and equipment contaminated by feces, secretions or blood must be cleaned and disinfected immediately by personnel in charge of the patient. All laundry and waste material should be properly cleaned or disposed of and contaminated surfaces cleaned and disinfected. A separate mop and bucket must be provided for the isolation area. Discarded protective wear, treatment materials, and waste matter should be discarded in waste bags and directly taken to the trash. Label all laundry from the area for disinfection. Any visitors to the isolation unit must adhere to all infection control policies and procedures. Similar protocols and procedures are employed for patients that are immune deficient. These patients should be housed in the general ICU area, but separated from other animals. They should not be placed in the isolation unit utilized for contagious disease. Reverse barrier isolation techniques should be used including dedicated nursing personnel that adhere to strict hand‐washing procedures and protective wear. Minimal movement within the common area will minimize patient exposure to pathogens from the general hospital population or personnel. There are many reasons why antibiotic therapy can fail to improve the clinical condition or outcome in a critical patient (Box 14.4). When this occurs, the history, physical examination findings, laboratory data, and advanced diagnostics should be reexamined. Different types of bacteria, such as anaerobes or Mycoplasma, should be considered along with the possibility that the pathogen is not bacterial at all. The wrong antibiotic may have been chosen or the wrong drug or dose administered to the specific patient. Consider drug dose miscalculation, inappropriate administration, and failure of the drug delivery or penetration as causes of poor response. Antibiotics are ineffective within abscesses or septic exudates and medical therapy is no replacement for appropriate debridement and drainage. Unfortunately, bacteria can rapidly develop antibiotic resistance to even the most appropriate drug regimen. Inappropriate drug choice, dosage, dosing interval, length of therapy, and repeated antibiotic exposure all contribute to development of antibiotic resistance. Nosocomial and superinfections warrant prompt and aggressive intervention. Patients with complicating co‐morbidities such as immune deficiencies, diabetes mellitus, hyperadrenocorticism, etc. will be at increased risk from these infections.
White blood cells, immune status, and antimicrobial stewardship
Introduction
, cytokine release;
, activation; NK, natural killer cell; PMN, polymorphonuclear cell; WBCs, white blood cells.
Diagnostic and monitoring procedures
History and physical examination
Point of care testing
Clinicopathological testing
Site
Technique
Tissue aspirate
Clean intact skin, air dry, 22 G needle aspirate from several areas, remove needle with hemostat, discard needle, and recap
Swabs (eyes, ears, uterus ONLY)
Moisten swab with preservative‐free solution, collect without touching skin, rotate swab over 1 cm area for 4 sec, place in transport sleeve
Skin/wound
DO NOT use swabs, DO NOT collect pus, instead aspirate or collecte macerated tissue or biopsy. For superficial skin, scrape cleaned skin and allow to air dry. Transport in liquid media
Bone
Collected aseptically, keep moist with saline
Drain site
Treat as contaminated wound, DO NOT culture tube as biofilm may misrepresent sample, collect tissue at site of infection
Urine
Cystocentesis after surgical scrub, keep sample cold and submit within 24 hours
Blood culture
Using liquid media (1 part blood:10 parts media), collect from at least three sites at three different time points, ideally when a fever is present
Cerebrospinal fluid/joints
Collect into blood culture media, collect aseptically.
Gastrointestinal tract
Fecal specimens may be collected for Campylobacter, Shigella, Salmonella, Clostridium, Yersinia, Vibrio, Aeromonas, and some E. coli spp.
SIRS criteria dogs
SIRS criteria cats
The leukogram
Cell type
Description
Function
Dysfunction
Lymphocytes
Large single pink‐staining nucleus. Produced in lymph nodes and other lymphoid tissues. Include B‐cells, T‐cells, memory cells, plasma cells, and natural killer cells
Responsible for cell‐mediated and humoral immunity
Severe combined immunodeficiency. Congenital disorders. May have normal lymphocyte numbers with abnormal function
B‐cells
Responsible for humoral immunity. Produce immunoglobulins after differentiation into plasma cells. 12% of total lymphocytes. Mature and proliferate in lymph nodes
Produce antibodies. Combat pyogenic bacteria. Prevent blood‐borne infections. Neutralize toxins. Usually require T‐cell assistance to respond to antigen. Can also recognize antigen directly through surface Ig. Responses are antigen specific while effects are mainly through complement
T‐cells
Helper T‐cells
CD4+
CD8+
Responsible for cell‐mediated immunity; 70–80% of total lymphocytes
Recognize antigen within presenting cells. Release cytokines to control development of immune response
Recognize and kill cells infected with virus
Especially important against intracellular organisms including protozoa and fungi. Role in graft rejection and immune surveillance of neoplasia. Long‐lived memory cells. Activity by cytokine production
Memory cells
T‐ and B‐cells that are antigen specific or Ig secreting
Part of secondary immune response. Recruited after second exposure to Ag
Plasma cells
These are fully differentiated B‐cells. Role in innate and adaptive immune response
Secrete antibodies. Kill virus‐infected cells. Role in antigen presentation
Natural killer cells
Also called large granular lymphocytes. Part of lymphoid system, have no differentiating cell surface markers
Efficacy against virus‐infected and neoplastic cells
Monocytes/
macrophages
Blood‐borne monocytes will replenish tissue‐specific macrophage populations. These are long‐lived cells with widespread distribution. Stem cell differentiation via monocyte‐colony stimulating factor
Responsible for phagocytosis and killing of microorganisms; activation of T‐cells, innate immunity, and antigen presentation. Surface receptors for Ig and activated complement allow cells to take up immune complexes. Major source of colony stimulating factors and cytokines
Dendritic cells
Found mainly in lymphoid tissue
Function as antigen‐presenting cells. Initiate adaptive immune response. Most potent stimulators of T‐cell activation
Mast cells
Allergic reactions
Release granules containing histamine, leukotrienes, chemokines, and cytokines
Neutrophils
(granulocytes)
Also called polymorphonuclear cells (PMNs). Short‐lived (12 h in blood, 12 h in tissues). Circulating and marginated pools. Granulocyte‐colony stimulating factor triggers cell differentiation during production in bone marrow
Responsible for phagocytic clearing of bacterial infections. Role in innate immunity. Circulating PMNs interact with endothelial surface via selectins, then form firm adhesion with integrins for final margination at site of infection or inflammation
Neutropenia can be caused by increased margination, peripheral destruction or decreased bone marrow production. Inappropriate neutropenia may indicate immune dysfunction or incompetence in critically ill patients. Lysosomal storage disorders can cause dysfunction. Congenital disorders include Chédiak–Higashi Syndrome and Pelger–Hüet anomaly
Eosinophils
(granulocytes)
Cells with double‐lobed nucleus, orange cytoplasmic granules containing toxic compounds. Interleukin‐5 triggers stem cell differentiation
Kill antibody‐coated antigens through degranulation. Prominent in allergic inflammation
Basophils
Blue granules containing toxic and inflammatory compounds. Stem cell factor triggers differentiation
Cell‐killing cells. Important in allergic reactions and parasitic infections
Test
Reference range
Units
Canine
Feline
WBC
4.9–17.6
3.9–19.0
K/μL
RBC
5.39–8.70
7.12–11.46
M/μL
HGB
13.4–20.7
10.3–16.2
g/dL
HCT
38.3–56.5
28.2–52.7
%
MCV
59–76
39–56
fL
MCH
21.9–26.1
12.6–16.5
pg
MCHC
32.6–39.2
8.5–37.8
g/dL
Reticulocyte
10–110
3–50
K/μL
Platelet
143–448
155–641
K/μL
Neutrophil
2940–12670
2620–15170
/μL
Lymphocyte
1060–4950
850–5850
/μL
Monocyte
130–1150
40–530
/μL
Eosinophil
70–1490
90–2180
/μL
Basophil
0–100
0–100
/μL
Condition
WBC
Seg
Band
Lymph
Mono
Eos
Acute inflammation
↑
↑
↑
↓, or no change
Variable
Variable
Chronic inflammation
↑, or no change
↑, or no change
↑, or no change
↑, or no change
↑
Variable
Overwhelming inflammation
↓, or no change
↓, or no change
↑
↓, or no change
Variable
Variable
Excitement leukocytosis
↑
D – ↑
C – ↑, or no change
No change
D – no change
C – ↑
No change
No change
Stress leukogram
↑
↑
No change
↓
↑, or no change
↓, or no change
White blood cell lines
Increased WBCs
Decreased WBCs
NeutrophiliaInflammation
Infection
Tissue necrosis, ischemia
Corticosteroids (endogenous or exogenous)
Rebound neutrophilia
Hemorrhage or hemolysis
Primary neoplasia (myelogenous leukemia)
Functional defects affecting adhesion
NeutropeniaAcute, severe inflammation
Increased peripheral destruction
Immune‐mediated destruction
Decreased bone marrow production
Hereditary (Gray Collies)
Lymphocytosis
Lymphopenia
Reactive lymphocytes
Hypoadrenocorticism
Epinephrine induced (cats)
Glucocorticoid deficiency
Lymphoid leukemia
Stress response (750–1500 K/μL)
Acute infection
Lymphedema
Monocytosis
Monocytopenia
Inflammation
Immune‐mediated hemolytic anemia
Tissue necrosis
Foreign body reactions
Pyogranulomatous inflammation
Organisms such as fungi, filamentous bacteria, Mycobacteria, Pythium, Toxoplasma
Erythrophagocytosis
Hemophagocytic syndrome
Monocytic neoplasia
Chronic infection
Bone marrow disorder
Folate and B12 deficiencies
Endotoxemia
Chemotherapy
Eosinophilia and basophilia
Eosinopenia and basopenia
Allergic reactions
Parasitic infections
Inflammation
Ongoing systemic hypersensitivity
Hypoadrenocorticism
Neoplasia
Paraneoplastic diseases
Systemic mastocytosis
Idiopathic hypereosinophilia
Esosinophils
Basophils
Neutrophils
Lymphocytes
Monocytes
Eosinophils and basophils
Bacterial culture and susceptibility testing
Diagnostic imaging
Monitoring procedures
Antimicrobial stewardship
Patient factors
Diagnostic factors
Fever, hyperthermia
Severe prolonged hypotension
Severe gastrointestinal signs
Open wound
Hot, swollen joints, tissues
Bone pain on deep palpation
Acute abdominal pain
Purulent exudate
Abscessation or pustules
Cough
Vasculitis
Enlarged painful prostate
Sudden‐onset or new heart murmur
Leukocytosis with left shift
Leukopenia
Hypoglycemia
Thrombocytopenia
Hypoalbuminemia
Bacteria seen on cytology (tissue aspirate, sputum, bronchial fluid, urine, purulent exudate, abdominal fluid, etc.)
Bacteriuria/pyuria
Hyperbilirubinemia
Abnormal coagulation
Free abdominal gas on radiographs
Positive bacterial culture results
Enlarged uterus on imaging
Glucose lower in abdominal fluid than in blood
Cardiac valvular lesions (with fever)
Infection site/organ
Common pathogens
Skin
Staphylococcus pseudointermedius is the primary organism in pyoderma. Secondary bacteria include Escherichia coli, Proteus, Pseudomonas [34]
Urinary tract
Escherichia coli, Staphylococcus, Streptococcus, Enterococcus, Proteus, Pseudomonas, Klebsiella, Enterobacter, Corynebacterium, and more [35,36]
Gastrointestinal
Small bowel: E. coli, Staphylococcus, Clostridium perfringens, Bacteroides, Salmonella, Shigella, Campylobacter jejuni, H. pylori
Hepatobiliary
Escherichia coli, Enterococcus, Staphylococcus pseudintermedius, Streptococcus, Klebsiella pneumoniae, Enterobacter, Pseudomonas, Citrobacter, Clostridium spp., Propionibacterium acnes, Actinomyces, Corynebacterium, Bacteroides, Peptostreptococcus and others [37]
Reproductive
Pyometra: E. coli is the most common, others include streptococci, staphylococci, Proteus, Klebsiella, Serratia, Salmonella, Pseudomonas, Clostridium [38],Pasteurella, and Moraxella [36]
Prostate: E. coli, Staphylococcus, Streptococcus, Klebsiella, Proteus, Mycoplasma, Pseudomonas, Enterobacter, Pasteurella, Hemophilus [39]
Mastitis: Staphylococcus, Escherichia coli, Streptococcus, Klebsiella
Central nervous system
Staphylococcus, Streptococcus, Pasteurella, E. coli, Klebsiella spp., Proteus spp., Salmonella spp., Actinomyces spp., Nocardia spp. Anaerobes have been identified as well [40]
Respiratory (pneumonia)
Bordatella, Mycoplasma, Streptococcus, E. coli, Klebsiella, Pseudomonas spp., Yersinia pestis, Mycobacteria, Pasteurella, Neisseria, Staphylococcus, Moraxella [41]
Respiratory (pyothorax)
Dogs: Bacteroides, Pasteurella, Peptostreptococus, Fusobacterium, E. coli, Actinomyces, Streptococcus canis, Staphylococcus pseudintermedius, Prevotella, Nocardia nova
Cats: Pasteurella, Bacteroides, Fusobacterium, Clostridium, Peptostreptococcus, Actinomyces, Porphyromonas, Prevotella
Cardiovascular (endocarditis)/hematogenous
Staphylococcus pseudintermedius or coagulase‐positive spp., Streptococcus, Enterococcus, Corynebacterium, E. coli, Salmonella, Enterobacter, Klebsiella pneumoniae, Pseudomonas aeruginosa (cats), Proteus (cats), Bartonella, Clostridium perfringens, Propionibacterium acnes, Bacteroides spp., Fusobacterium spp. [42]
Bone/joint
Osteomyelitis: primarily Staphylococcus; other organisms may include E. coli, Klebsiella, Pasteurella, Serratia, Proteus as well as some anaerobic bacteria: Bacteroides, Fusobacterium, Clostridium and others [36]
Drug class
Mechanism of action (MOA) and spectrum of activity
Adverse effects
Penicillins
MOA: binding to penicillin‐binding proteins to cross‐link peptidoglycans. This inhibits bacterial cell wall synthesis. Bactericidal
GI distress, hypersensitivity reactions
Spectrum: Gram +, Gram − and anaerobic bacteria. Ticarcillin combinations will have increased activity against Pseudomonas
Amoxicillin
20–30 mg/kg IV, IM, SC q 6–12 h (d)
Ampicillin
20–40 mg/kg IV, IM, SC q 6–8 h (d,c)
Ampicillin‐sulbactam
22–30 mg/kg IV q 6–8 h (d,c)
Ticarcillin‐clavulanate
50 mg/kg IV q 6–8 h (d,c)
Cephalosporins
MOA: binding to penicillin‐binding proteins to cross‐link peptidoglycans. This inhibits bacterial cell wall synthesis. Bactericidal
GI distress, hypersensitivity reactions
Spectrum: varies by generation of drug. Not interchangeable. General rule of thumb is increased gram‐negative coverage with higher number generations. Anaerobic coverage varies
Cefazolin
22–30 mg/kg IV, IM, SC q 6–8 h (d,c)
First generation
Cefoxitin
30 mg/kg IV q 6–8 h
Second generation
Ceftazidime
20–30 mg/kg IV q 6–12 h (d,c)
Third generation
Cefovecin
8 mg/kg SC q 7 days
Third generation
Cefotaxime
25–50 mg/kg IV q 6–8 h (d,c)
Third generation
Carbapenems
MOA: binding to penicillin‐binding proteins to cross‐link peptidoglycans. This inhibits bacterial cell wall synthesis. Bactericidal
GI distress, hypersensitivity reactions. These are broad spectrum. Recommend use only if indicated by culture and sensitivity results. Cilastatin is used to slow renal drug clearance
Spectrum: Gram +, Gram − and anaerobic bacteria. Not effective against methicillin‐resistant organisms
Imipenem‐cilastatin
5–10 mg/kg IM, IV q 6–8 h (d,c)
Meropenem
8–12 mg/kg IV q 8–12 h (d,c)
Aminoglycosides
MOA: binding to 30S ribosomal subunit. Bactericidal
Spectrum: primarily Gram − organisms, some Gram +, aerobes only
Nephrotoxicity, ototoxicity, neuromuscular paralysis
PAE allows q 24 h dosing
Synergistic with beta‐lactams
Amikacin
15–30 mg/kg IV, IM, SC q 24 h (d), 10–15 mg/kg IM, SC q 24 h (c)
Gentamicin
6–10 mg/kg IV q 24 h (d), 6 mg/kg IV q 24 h (c)
Macrolides
MOA: reversible binding to 50S ribosomal unit. Cidal or static depending on organism
Spectrum: Gram +, Mycoplasma spp., Bartonella, Borrelia, Campylobacter, Chlamydia, Leptospira, Campylobacter. Anaerobes except Bacteroides
GI distress (esp. severe with erythromycin)
Concentration in macrophages will achieve high concentration at site of infection
Azithromycin
5–10 mg/kg IV q 24 h (d,c)
Erythromycin
10–20 mg/kg IV q 8–12 h (d,c)
Phenicols
MOA: inhibits 50S ribosomal unit. Bacteristatic
Spectrum: Gram +, Gram −, anaerobes, spirochetes, Rickettsia, Chlamydia, Bordatella, Mycoplasma, Hemobartonella
In patients: reversible and irreversible bone marrow suppression; GI distress. In human caretakers: risk of idiosyncratic irreversible bone marrow suppression
Chloramphenicol
25–50 mg/kg IV, IM, SC q 8 h (d), 15–20 mg/kg IV, IM, SC q 6–12 h (c)
Lincosamides
MOA: inhibit 50S ribosomal subunit, at a site separate from macrolides. Bacteriostatic
Spectrum: Gram + cocci and anaerobes, Toxoplasma gondii, Neospora caninum, Hepatozoon, Babesia. Concentrated in phagocytic leukocytes. May have cidal or static effect depending on concentration and site
GI distress, esophageal injury (oral administration)
Clindamycin
10–15 mg/kg IV q 12 h (d,c)
Tetracyclines
MOA: bind to 30S ribosomal unit. Bacteriostatic
Spectrum: Gram +, Gram −, Mycoplasma, Hemobartonella, Leptospirosis, Pasteurella, E. coli, Klebsiella, Salmonella
GI distress; collapse if administered quickly; esophageal injury (oral administration); discoloration of teeth in juvenile patients
Doxycycline
5–10 mg/kg IV q 12 h (d,c)
Fluoroquinolones
MOA: directly inhibits DNA synthesis by action on topoisomerases
Spectrum: primarily Gram −, some Gram +
GI distress, cartilage defects, blindness (cats)
Long postantibiotic effect
Enrofloxacin
10–15 mg/kg IV q 24 h (d), 5 mg/kg IV q 24 h (c)
Glycopeptides
MOA: inhibit cell wall synthesis. Bactericidal
Spectrum: Gram + aerobes and anaerobes. Resistant strains of Staphylococcus spp., Streptococcus spp., anaerobes
Histamine release (hypotension tachycardia), potentiates nephrotoxicity, GI distress
Ethical use in veterinary patients remains under debate. Use only as last resort. Typically superior choices exist
Vancomycin
15 mg/kg IV q 8 h (d), 10–15 mg/kg IV q 8–12 h (c)
Nitroimidazoles
MOA: metabolite inhibits nucleic acid synthesis. Bactericidal
Spectrum: metabolite produced primarily in anaerobic cells, so major effect is on anaerobic bacteria. Clostridium difficile. Gram +, Gram −, anaerobes, some protozoa. Some antiinflammatory effect
Neurotoxicity (central vestibular signs, seizures, cerebellar dysfunction); hepatotoxicity; urine discoloration
Metronidazole
10–15 mg/kg IV q 12 h (d,c)
Antifolates
MOA: block DNA and RNA synthesis by inhibition of purine metabolism. Bacteristatic.
Spectrum: Gram − staphylococci, some streptococci, Nocardia, Protozoa, Mycobacteria, Pneumocystis, unpredictable against streptococci, no activity against enterococci or obligate anaerobes
Hypersensitivity reactions; bone marrow suppression; dysuria; inhibition of thyroid hormone synthesis; keratoconjunctivitis sicca; idiosyncratic reactions; polyarthropathy
Trimethoprim‐sulfamethoxazole or sulfadiazine
30 mg/kg IV q 12 h (d,c)
Antibiotic selection
Indication
Drugs
Note
Peritonitis
Gentamycin + clindamycin
Broad‐spectrum activity
Cefuroxime + metronidazole
Broad‐spectrum activity
Coliform meningitis
Trimethoprim + sulfamethoxazole
Synergistic with good cerebrospinal fluid penetration
Cryptococcal meningitis
Amphotericin + flucytosine
Synergistic, decreased toxicity
Severe undiagnosed infection
Beta‐lactam + aminoglycoside
Broad spectrum, synergistic
Cefoxitin + clindamycin
Broad spectrum, synergistic
Perioperative antibiotics
Hospital antibiotic surveillance
Creating an antibiotic protocol
The antibiogram
Nosocomial infections
Bacterial resistance
Hygiene and environmental surveillance
Patient isolation
Failed response to antibiotic therapy
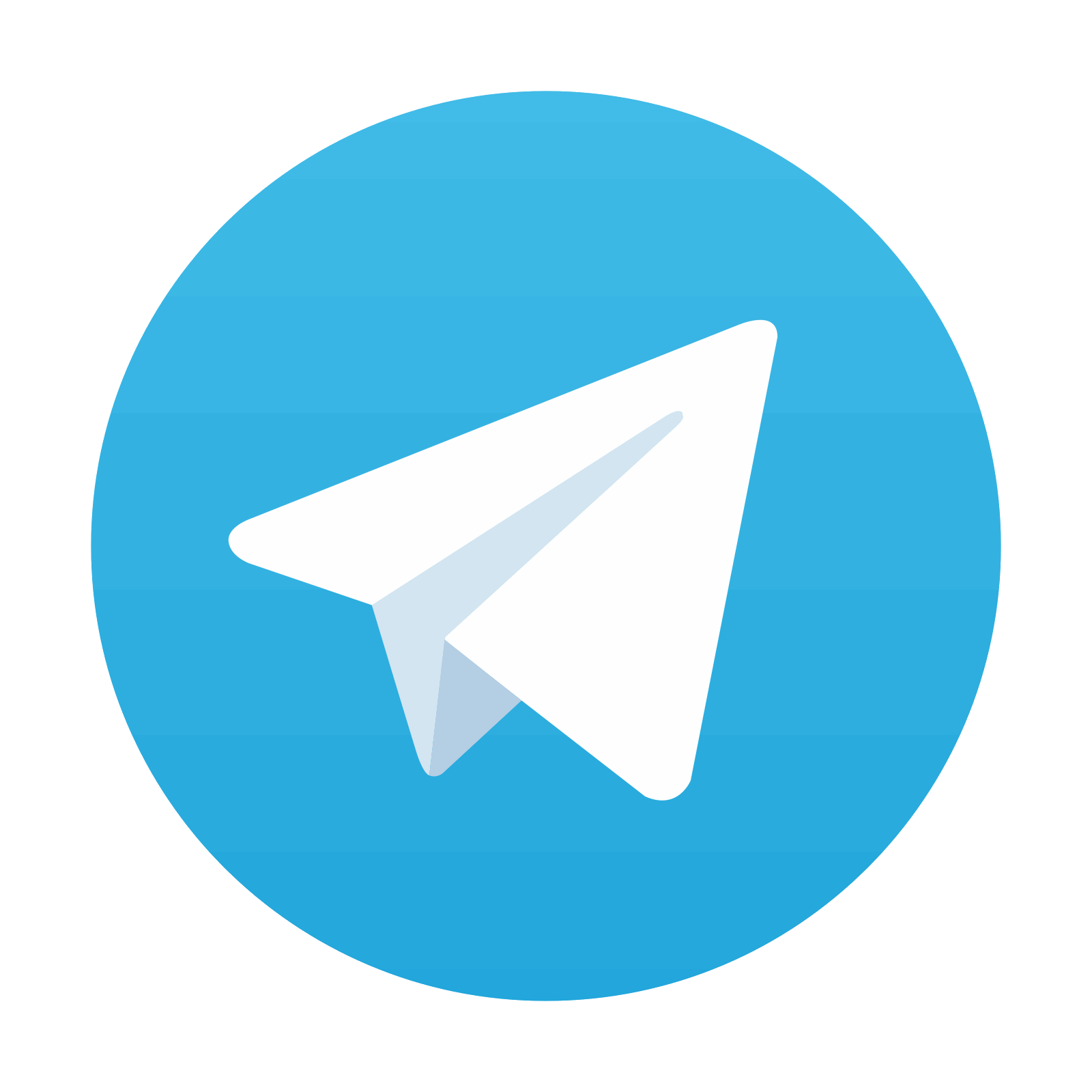
Stay updated, free articles. Join our Telegram channel

Full access? Get Clinical Tree
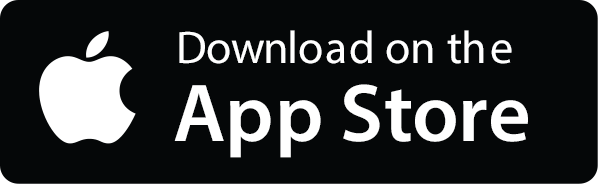
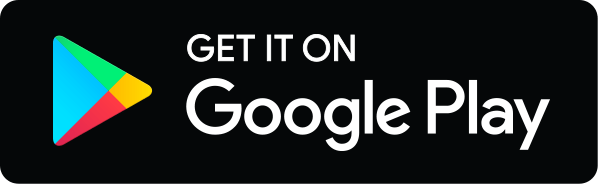