CHAPTER 13 Lee Herold DoveLewis Emergency Animal Hospital, Portland, Oregon The urinary tract consists of the kidneys, ureters, urinary bladder, and urethra. Insult to this system can be attributed to prerenal (blood flow to the kidneys), intrinsic renal (renal parenchyma) or postrenal (ureteral, urinary bladder or urethral) lesions (Table 13.1). Many critically ill patients will have a combination of insults [1,2]. Table 13.1 Selected causes of prerenal, renal, and postrenal azotemia. C, canines; F, felines. The responsibilities of the kidneys are diverse and proper function is required for homeostasis [3]. Renal functions include but are not limited to the maintenance of water, electrolyte and acid–base balance, production of hormones (such as erythropoietin and vitamin D3), excretion of toxic metabolites, and production of energy through gluconeogenesis [3]. The functioning unit of the kidney is the nephron, which consists of a tuft of capillaries called the glomerulus surrounded by Bowman’s capsule that leads to the renal tubules (Figure 13.1) [1,3]. The glomerulus and Bowman’s capsule are collectively referred to as the renal corpuscle which is the site of plasma ultrafiltration [1,3]. The volume of ultrafiltrate produced at the glomerulus is dependent on hydrostatic pressures within the glomerular capillaries, determined by systemic blood pressure and by the relative degree of dilation or constriction of the afferent and efferent arterioles. The pressure within the glomerular tuft is often referred to as transglomerular capillary pressure. Bowman’s capsule is composed of interdigiting cells called podocytes, surrounded by a specialized glomerular basement membrane. The degree of interdigitation of podocytes and the negative electric charge of the glomerular basement membrane serve to exclude plasma proteins from being ultrafiltered and lost in the urine in the healthy patient. Figure 13.1 The functional unit of the kidneys: a nephron. Diseases that limit renal blood flow or lower transglomerular capillary pressure can contribute to alterations in volume of the glomerular filtrate or the glomerular filtration rate (GFR) [1–3]. Additionally, pathological changes in the glomerular basement membrane can lead to plasma components such as albumin being leaked in the urine. The renal tubules are divided into proximal tubules, the loop of Henle, distal convoluted tubules, and connecting segment and collecting tubules. These tubular segments are differentiated by their physiological function [3]. The proximal tubule consists of convoluted and straight segments and is the site of active resorption of sodium and isoosmotic resorption of water and filtered solutes. Distinguishing characteristics of the proximal tubular cells include extensive surface area for resorption provided by brush border microvilli, large quantity of mitochrondria providing energy for the high resorption function, and intercellular junctions that allow paracellular transport. The loop of Henle is the site of sodium and chloride resorption in excess of water. Its ability to absorb sodium and water and change the osmolality of urine is due to different permeability characteristics of cells along the loop as well as the countercurrent exchange mechanism. The distal convoluted tube, connecting ducts, and collecting tubules are the final sites of fine and qualitative changes to the urine. The distal nephron is responsive to hormones such as parathyroid hormone and calcitriol to maintain calcium homeostasis, and antidiuretic hormone and aldosterone for fine adjustments in sodium, potassium, and water handling [3]. Azotemia is defined as an increase in serum or blood urea nitrogen (BUN) and creatinine while uremia is the clinical syndrome (pathological manifestations) that develops from severe or prolonged renal azotemia [1,2]. Azotemia can be prerenal, renal or postrenal in origin, with the differentiation being important when planning the stabilization, diagnostic, and therapeutic plan for the critical patient. Acute kidney injury (AKI) implies an abrupt deterioration in renal function [1,2,4]. Chronic kidney disease (CKD) implies kidney damage that has been present for at least three months and is common in our veterinary species [1]. Urinary tract problems can be the presenting concern in the small animal ICU patient, can develop as a consequence of an unrelated systemic illness, or can complicate the progression or response to therapy of an unrelated condition [1,2,4]. The presence of CKD can also cause the animal to be more susceptible to further renal insult, whether prerenal, intrinsic renal or postrenal in origin. A systematic approach to the diagnosis and monitoring of urinary tract problems in the critically ill veterinary patient is essential for early detection of alterations and to provide a basis for early intervention. An abnormality in any portion of the urinary tract can potentially have life‐threatening consequences. Evaluation begins with a thorough history and physical examination. Rapid initial assessment is available with cage‐side point of care (POC) testing and can identify many important metabolic alterations. This is followed by more thorough clinicopathological testing and imaging. The diagnostic approach to urinary tract disorders is determined by the specific risk characteristics of the patient. Careful patient monitoring is required throughout the diagnostic and treatment period. Clinical history is essential to the diagnosis and assessment of risk for renal injury. The signalment can direct diagnostic efforts to breed‐related (such as Fanconi’s syndrome in Basenjis, Samoyed hereditary glomerulopathy) or congenital and hereditary disorders such as polycystic kidneys. Intact male and female patients may show signs of urinary tract disorders secondary to vaginal, uterine or prostatic disease. Past medical history, including preexisting renal disease, as well as chronic medication history is assessed. Queries are particularly made regarding any medications that may alter renal hemodynamics or have the potential to be nephrotoxic, such as nonsteroidal antiinflammatory drugs (NSAIDS), angiotensin converting enzyme (ACE) inhibitors (ACEi), antihypertensive drugs, and chemotherapeutic agents. Potential exposure to environmental nephrotoxins, including lilies (toxic to cats), raisins or grapes (toxic to dogs), ethylene glycol or dietary supplements such as alpha‐lipoic acid, vitamin D‐containing toxins, supplements or medications should be identified. Environmental and housing history may highlight the risk of infection with leptospirosis [1,2]. Patients with uremia may have shown signs of hyporexia to anorexia, nausea, vomiting, weight loss, and blood in vomitus (suggestive of gastrointestinal (GI) ulcerations) [1,2,5,6]. The history pertaining to water intake (polydipsia or adipsia) and urination of the animal is essential. Information regarding the estimated amount of urine passed with each effort, the frequency of urination and presence of pain or straining during urination provides essential clues to the underlying disorder. Pollakiuria (increased frequency of urination), polyuria (increase in quantity of urine), and oliguria (reduced urine quantity) are signs of urinary tract problems that the owner has likely observed [7]. The appearance of the urine may direct diagnostic efforts as well; cloudy urine suggests infection, blood in the urine is possible with stones, trauma, hemoglobin or infection, and dark‐colored urine suggests bilirubin, hemoglobin or myoglobin [7,8]. Establishing a baseline for temperature, pulse, and respiratory rate can provide important information regarding any systemic effects of urinary tract disorders. A high body temperature may be present with infectious or inflammatory causes of renal dysfunction. Hypothermia is a common consequence of endogenous renal toxins causing a reset of the thermoregulatory center. Heart or pulse rate can be affected by poor perfusion (tachycardia in dogs, normal rate or bradycardia in cats) or significant hyperkalemia (bradycardia in dogs, tachycardia or bradycardia in cats) secondary to renal failure or urinary tract obstruction or disruption. Severe metabolic acidosis can cause Kussmaul type breathing, while uremic pneumonitis or fluid overload can cause tachypnea and labored breathing. Physical evaluation of effective circulating volume includes heart rate, pulse quality, mentation, mucous membrane color, and capillary refill time to allow stratification of patients into the categories of compensatory, early decompensatory, and late decompensatory shock [9]. The physical assessment of hydration will include skin turgor, mucous membranes, and corneal moisture [9]. Additional physical examination findings that may indicate the presence of renal disease include oral mucosal ulcerations, decreased body condition in patients with chronic renal failure, and uremic odor to the breath. Special focus should be placed on renal palpation during abdominal palpation to note renal size, symmetry, or the presence of pain. Urinary bladder palpation should be performed to detect pain or a turgid urinary bladder that may suggest obstructive disease. If the urinary bladder is empty of urine and urinary stones are large, they may be palpable on transabdominal palpation. Digital rectal examination can detect some urethral masses, stones, or urethral distension. In male dogs and cats, the penis should be extruded to evaluate for discharge, discoloration, penile or distal urethral masses. In female dogs, digital vaginal exam can be performed if the clinical history warrants it. Fundic examination should be performed to evaluate for signs of hypertensive retinopathy, including tortuous retinal vessels, retinal hemorrhage, or detachment [1,2]. The initial POC testing begins with the minimum database consisting of packed cell volume (PCV), total protein (TP), blood urea nitrogen (BUN), glucose, electrolytes, blood gas for acid–base analysis, coagulation profile with buccal mucosal bleeding time (BMBT), and urinalysis (UA). Hemoconcentration may indicate dehydration. Anemia indicated by a low PCV can be a result of bleeding or CKD. Hypoproteinemia (low TP) can be present due to albumin loss through the urine (glomerular disease) or concurrent liver disease. Urea nitrogen is a byproduct of endogenous and exogenous protein metabolism by the liver. Since urea nitrogen is freely filtered from the blood at the glomerulus, the BUN can be useful as a marker of renal glomerular filtration rate (GFR). However, the BUN level will also elevate due to a reduction in renal blood flow in an adequately functioning kidney. Extrarenal influences should be considered when renal function and renal blood flow are adequate [1,2,10]. The BUN can also be elevated due to an increased rate of urea production (postprandial from dietary protein) or GI bleeding. A low BUN warrants assessment of hepatic function. The blood glucose is evaluated and correlated with the presence or absence of urine glucose. The renal threshold for glucose in the cat is 300 mg/dL (17 mmol/L) and 180 mg/dL (10 mmol/L) in dogs. Glucose found in the urine when the blood glucose is below the renal threshold localizes the problem to a renal proximal tubular disorder [7]. Acid–base and electrolyte abnormalities in patients with renal failure contribute to the clinical manifestations of uremia and are potential therapeutic targets. A high anion gap metabolic acidosis is the most common acid–base derangement in patients with renal failure [2]. However, metabolic alkalosis may be present in patients with renal failure that are experiencing severe vomiting. The acid–base status should be monitored at least daily in any critically ill patient (see Chapter 7) [2]. Serum electrolytes may be abnormal due to kidney failure or postrenal obstruction or disruption. Of emergent interest is serum potassium since it can increase with a relative decrease in urine output. The presence of hyperkalemia warrants evaluation of the electrocardiogram and urine output, and may require immediate intervention to stabilize myocardial conduction. In contrast, patients with chronic renal failure often have low potassium levels due to chronic polyuria, chronically decreased potassium intake and total body potassium depletion (see Chapter 6) [11]. Hypercalcemia can be the cause of acute renal failure or the result of chronic renal failure. The clinical signs associated with hypercalcemia are dependent on the rate and magnitude of rise in serum calcium. Acute, severe increases or long‐standing increases in serum calcium are more likely to result in kidney injury and resultant clinical signs of polyuria, polydipsia, uremia, and lab‐detected azotemia. Differentials for hypercalcemia include hyperparathyroidism, neoplasia including osteolytic processes, hypoadrenocorticism, vitamin D toxicities, some types of granulomatous infections, and chronic renal failure [1,2]. Mild increases in serum calcium do not require specific therapy except to treat the underlying cause. However, severe hypercalcemia resulting in azotemia may require specific interventions, discussed under the acute kidney injury section below. Mild hypocalcemia is a common result of chronic renal failure as renal secondary hyperparathyroidism progresses [11,12]. Hypocalcemia can occur with severe hypoalbuminemia in patients with protein‐losing nephropathies. Clinical signs of hypocalcemia including tremors, tetany, and seizures are uncommon in patients with hypocalcemia associated with renal insufficiency (see Chapter 6). The BMBT measures the ability to form an initial platelet plug after capillary injury with a lancet. The components of the coagulation system assessed during BMBT measurement are the endothelial and vascular response to injury, including contribution to the platelet plug by platelets and functional von Willebrand factor. The BMBT can be prolonged with severe thrombocytopenia so results of BMBT should be evaluated in conjunction with a platelet count. Uremic coagulopathy is characterized by platelet dysfunction and BMBT may be useful as a screening tool for uremic coagulopathy (see Chapter 9). The UA provides results that are integral in the diagnosis and monitoring of urinary tract disorders [4]. The testing is best performed on urine that is collected by aseptic methods of cystocentesis or urinary bladder catheterization. The gross character of urine can indicate the presence of hematuria/hemoglobinuria (red urine), bilirubinuria (bright yellow or greenish urine), methemoglobinuria or myoglobinuria (brown to black urine) [7,8]. Differentiating from hemoglobinuria and myoglobinuria can be challenging but because hemoglobin binds tightly to haptoglobin and does not clear as readily from the serum as myoglobin, a patient with a dark pigmented urine and a red coloration to the serum is more likely to have hemoglobinuria, whereas a patient with dark pigmented urine but with clear serum is more likely to have myoglobinuria. Evaluation of urinary and serum pigments in the face of urinary tract disease is important because excretion of an excess of serum pigments can contribute to nephropathy and renal failure [7,8]. Isosthenuria, demonstrated by a urine specific gravity of 1.008–1.020, concurrently with elevation of the BUN and creatinine, is the classic diagnostic criterion for intrinsic renal disease. Urine that is highly concentrated (>1.035) may indicate a prerenal component to the azotemia. Hyposthenuria (<1.008) can narrow the differential list to problems such as psychogenic polydipsia, iatrogenic medullary washout, or nephrogenic or central diabetes insipidus. The UA results beyond urine specific gravity are valuable in determining some etiologies of intrinsic renal insult. The urinary dipstick has test pads for qualitative or semiquantitative assessment for urine protein, glucose, bilirubin, red cells, and leukocytes. The finding of glucosuria without serum hyperglycemia can suggest renal proximal tubular injury [8]. The presence of proteinuria with noninflammatory urinary sediment usually localizes the problem to the glomerulus and is further tested with the urine protein:creatinine ratio [13,14]. Examination of the urine sediment for the presence of casts, crystals, cells, and bacteria provides a reflection of the urine contained in renal tubules and the urinary bladder. The presence of inflammatory cells combined with bacteria supports urinary tract infection and possibly pyelonephritis (white cell casts). Urine cytology can be diagnostic of urinary tract neoplasms if there is exfoliation of neoplastic cells. The finding of crystalluria may assist in the diagnosis of urolithiasis. The presence of calcium oxalate crystals (octahedral or envelope shaped) can be a normal finding in dogs or cats but may also be the result of ethylene glycol toxicity, or indicative of calcium oxalate urolithiasis. Triple phosphate crystals, also called magnesium ammonium phosphate, or struvite crystals (“coffin lid” shaped) can be present in alkaline urine associated with stone formation or bacterial urinary tract infections. Ammonium biurate crystals (yellow‐brown “thorn‐apple” shaped) can be found with portosystemic shunts. Ammonium biurate crystals may also be the result of a genetic defect of the urate transporter, a finding in some normal Dalmations and English Bulldogs [7]. Urinary casts are composed of a matrix of Tamm–Horsfall protein secreted by renal tubular cells, which often contain imbedded cells or cellular debris. Casts mirror the lumen of renal tubules, and are easily identified on urinalysis. Urinary casts are categorized as cellular (red blood cell, white blood cell, tubular cell), granular, waxy or hyaline. Cellular, granular, and waxy casts represent different states of breakdown of the cellular component imbedded in the cast mucoprotein matrix. Cellular casts contain intact and discernible cells including red blood cells, renal tubular epithelial cells or white blood cells. The cells within granular casts have partially broken down, contributing to the granular appearance. The cells within waxy casts have disintegrated and are not discernible, contributing to the waxy appearance. Small quantities of hyaline or waxy casts may be a normal finding on urinalysis. The presence of cellular or granular casts represents tubular pathology. Tubular necrosis and tubular cell sloughing will contribute to tubular casts. Severe hematuria with trauma, coagulopathy, or idiopathic renal hemorrhage may result in red blood cell casts. White blood cell casts may be seen in severe pyelonephritis [7]. In addition to being a diagnostic aid, urinalysis can also be a valuable monitoring tool for the ICU patient. A high urine specific gravity during fluid therapy indicates that dehydration is still present. A lower urine specific gravity during fluid diuresis is appropriate. A daily examination of the urine sediment for tubular casts is a sensitive indicator of tubular injury and is of particular utility when monitoring for nephrotoxicity associated with aminoglycoside or other drug therapy [1,2,4]. A complete blood count (CBC) and serum biochemistry should be performed in patients with azotemia. Red blood cell (RBC) levels should always be interpreted in light of the patient’s fluid balance. Chronic renal failure is associated with normochromic, normocytic nonregenerative anemia secondary to decreased erythropoietin production [11]. If GI bleeding is contributing to anemia, the red cells are typically microcytic and hypochromic because of iron deficiency. Chronic anemia of renal failure can be mild to moderate in severity (see Chapter 10). The presence of leukocytosis may indicate an infectious or inflammatory component to the urinary tract injury. The serum biochemistry results will assist in the diagnosis of renal failure and, when interpreted with urinalysis, may help with localization to specific parts of the kidney. Creatinine elevation is the most widely used clinical marker of renal function, providing the most rapid and readily available surrogate for GFR [10]. Because serum creatinine is influenced by fewer extrarenal factors than BUN, changes in creatinine can be interpreted as proportional to the degree of renal insult and a reflection of the GFR and rate of tubular flow [1,2,10]. The serum biomarker symmetric dimethylarginine (SDMA) has been evaluated in dogs and cats [15–17]. SDMA has been shown to have a linear and reciprocal relationship to GFR and may be more sensitive than serum creatinine for the detection of chronic kidney disease in cats [15–17]. When the BUN and creatinine are interpreted in conjunction with UA results (such as abnormal specific gravity, proteinuria, renal casts), they remain the standard for the diagnosis of renal failure [1,2,4,10,18]. Increases in serum BUN and creatinine during therapy may signify progressive or ongoing renal injury, may be a warning for the development of oliguria, or may be a marker of inadequate fluid therapy. Decreases in BUN and creatinine during therapy can indicate improved hydration, improved GFR and tubular flow, renal recovery or a combination of these factors [1,2,4,10]. Monitoring the trend of change of BUN and creatinine in hospitalized patients could facilitate early detection of AKI as well as assessing the response to treatment. Serum phosphorus levels are the result of dietary intake of phosphorus balanced with renal excretion. Hyperphosphatemia is common in patients with acute and chronic renal failure due to decreases in GFR [4]. However, postrenal obstruction or disruption can also cause hyperphosphatemia. Elevations in serum phosphorus can cause a decrease in ionized calcium through renal secondary hyperparathyroidism (rHPTH). Renal secondary hyperparathyroidism has been demonstrated to occur early in renal disease. Calcium‐phosphorus changes have been associated with an increased mortality in CKD in humans, cats, and dogs. When the total calcium and phosphorus product exceeds 60–70, there is an increased risk of malignant calcification of tissues [11]. Evaluation of hepatic function is important when assessing for problems that affect both renal and hepatic function (such as leptospirosis or life‐threatening hepatorenal syndrome). Hepatorenal syndrome is characterized by renal failure as a consequence of end‐stage cirrhotic liver failure. Evidence of hepatic failure such as hypoalbuminemia, alterations in serum cholesterol, low BUN, high blood ammonia, and prolonged clotting times, in association with evidence of renal failure, supports the hepatorenal syndrome diagnosis. Hypoalbuminemia may occur as a consequence of albumin loss secondary to glomerulopathies. Since antithrombin may also be lost through the diseased glomerulus, patients with severe hypoalbuminemia should be assessed for hypercoagulability or for evidence of thrombus formation. The presence of proteinuria with an active urinary sediment including the presence of red blood cells, white blood cells + bacteria on the initial urinalysis warrants submission of a urine sample for culture and susceptibility testing. The presence of proteinuria without a reactive sediment directs the submission of urine for a urine protein:creatinine (UPC) ratio to identify potential glomerular sources of urine protein [13,14]. Urinary tract imaging modalities from plain to contrast radiographs and abdominal ultrasound are complementary in the diagnosis and management of patients with urinary tract pathology. No single imaging technique is preferred as the gold standard of evaluation. Patients with renal failure often undergo several imaging modalities to fully evaluate the upper and lower urinary tract, and to assist in therapeutic recommendations [19]. Abdominal radiographs will allow assessment of the size and symmetry of the kidneys and retroperitoneal space, the size of the urinary bladder, the size and position of reproductive organs associated with the urinary tract and the location and shape of other abdominal organs. Canine kidneys will appear as bean‐shaped on radiographs and measure 2.5–3.5 times the length of the second lumbar vertebra. The right kidney is usually one‐half length cranial to the left kidney. Renal size in the cat as measured on the VD radiograph varies from 2.4 to 3.0 times the length of the second lumbar vertebral body (L2). However, it is fairly common for older cats (over 10 years of age) to have kidneys that range from 2.0 to 2.4 times the length of L2, without biochemical changes indicative of renal disease [19]. Abdominal radiographs remain the standard of care for evaluation of the presence of radiopaque urolithiasis (such as calcium phosphate, calcium oxalate, silicate, struvite) anywhere in the urinary tract. Urate and occasionally cysteine uroliths may be radiolucent [19]. Excretory urography is an intravenous (IV) contrast radiographic study that can provide a subjective assessment of renal function [20]. It also detects the presence of pyelectasia or hydronephrosis and can demonstrate urine leakage from the level of the kidneys to the urethra. Table 13.2 provides guidelines for performing urinary contrast studies. The risk of the procedure must be evaluated due to the potential for contrast‐induced renal failure from IV iodinated contrast agents. The patient undergoing the procedure must be well hydrated with adequate perfusion prior to the administration of any contrast agent [20]. Table 13.2 Urinary contrast studies. GFR, glomerular filtration rate; IV, intravenous; VD, ventrodorsal. Positive contrast and double‐contrast cystourethrograms can be performed to detect bladder and urethral lithiasis caused by radiolucent calculi (see Table 13.2). Positive contrast placed into the urinary bladder can also be used to demonstrate urinary bladder rupture (Figure 13.2). Urinary bladder wall thickness, mural and luminal masses, strictures and polypoid diseases can be highlighted with a double‐contrast cystourethrogram [21]. Figure 13.2 Lateral radiograph of a positive‐contrast cystourethrogram demonstrating abdominal extravesicular location of contrast medium in a patient with traumatic bladder rupture. There is no urethral leakage of contrast. At surgery, a large tear was found at the apex of the urinary bladder. The open arrow demonstrates the tip of the urinary catheter within the distal urethra that was used to inject the contrast medium. Abdominal ultrasound is used to detect radiolucent uroliths and assess renal architecture, perinephric tissues, and the retroperitoneal space. Changes within the urinary tract that may be seen on ultrasound include renal cysts, renal infarcts, renal parenchymal echogenic changes suggestive of toxic injury, infiltrative disease or neoplasia, renal pelvic dilation, ureteral dilation, urinary bladder wall thickness, bladder luminal masses, and the detection of free peritoneal or retroperitoneal fluid from urine leakage [19]. Table 13.3 provides imaging and abdominal fluid analysis criteria for the diagnosis of uroabdomen [22,23]. Monitoring the trends of change over time with ultrasound (such as renal pelvic size for pyelonephritis or ureteral dilation for obstructive disease) may demonstrate therapeutic success or structural deterioration which may necessitate more aggressive intervention, such as surgery. Table 13.3 Supportive and diagnostic criteria for uroabdomen. (S) is supportive of the diagnosis of uroabdomen; (D) is diagnostic for uroabdomen. Multiple criteria are usually combined to achieve a diagnosis. Computed tomography (CT) and magnetic resonance imaging (MRI) are planar imaging modalities that provide sensitive morphological evaluation of the kidneys and urinary bladder [19]. CT or MRI technology is limited by the requirement for anesthesia and their diagnostic benefits in urinary tract disease should be compared carefully due to the wide availability and utility of abdominal radiographs and ultrasound. When CT or MRI is chosen, images and diagnostic yield are complementary to the information obtained with radiographs and ultrasound. Video urethrocystoscopy allows for direct visualization of the vaginal, urethral, and bladder mucosa for diagnosis of many lower urinary tract abnormalities, including cystitis, ectopic ureter, urethral and bladder masses, urolithiasis, and urethral diverticula. Urethrocystocopy can be used to direct the collection of diagnostic samples including cytology, biopsy, and samples for culture of the lower urinary tract. Urethrocystocopy is used for interventional procedures such as intracorporeal lithotripsy for breakdown and removal of urinary bladder calculi [24]. Methods to measure GFR are feasible in clinical practice and include nuclear scintigraphy (using radionucleotides and gamma camera) and iohexol clearance measurements. Iohexol is an iodinated contrast agent that was found to be a suitable marker for GFR. After IV injection, iohexol is eliminated in the urine by glomerular filtration, with no tubular secretion or reabsorption. Plasma iohexol clearance can be calculated with collection and measurement of iohexol in serial blood samples. Laboratory quantitation of iohexol is required by liquid chromatography or x‐ray absorption [25]. Nuclear scintigraphy and iohexol clearance methods of GFR measurement, though accurate, are unable to assess ongoing changes in GFR, and with the time and equipment needed for results, this gives them little clinical utility over the endogenous creatinine clearance in the veterinary ICU [10]. While the standard in human medicine for measuring GFR is the iohexol clearance test, the endogenous creatinine clearance can provide a reasonable estimation. A decrease in clearance indicates a decrease in GFR [25]. When performed correctly, a creatinine clearance test provides the benefit of detecting approximately 20% decrease in renal function, compared to the 75% functional loss that is necessary before elevations in BUN and creatinine concentrations are detected. In order to increase the diagnostic accuracy of creatinine clearance for GFR assessment, this test should only be performed in well‐hydrated, nonazotemic animals. The procedure for performing and calculating the endogenous creatinine clearance is provided in Table 13.4. Inaccuracies with the endogenous creatinine clearance test are possible due to incomplete evacuation of the bladder, tubular secretion of creatinine in some male dogs, possible increased extrarenal secretion of creatinine (such as in the GI tract), and measurement of noncreatinine chromagens in the serum. Creatinine clearance is useful to rule in or out kidney disease in patients with polyuria and polydipsia but without azotemia [25]. Table 13.4 Endogenous creatinine clearance. The fractional excretion of urinary electrolytes is the fraction of the filtered electrolyte that is then excreted in urine. This urine parameter has been used to assess the appropriateness of renal electrolyte handling by the kidney and aids in localizing the cause of renal azotemia in humans. The fractional sodium excretion is used most commonly [10]. Fractional excretion is easy to measure clinically with spot urine and serum electrolyte measurements normalized by simultaneous urine and serum creatinine measurement [26]. The formula is: where FE = fractional excretion, Na = sodium, Cr = creatinine. In humans, the results are used to help differentiate prerenal disease (FE <1%) from acute tubular necrosis or other kidney disease (FE >2–3%) [10,26]. Interpretation of fractional sodium excretion in veterinary medicine is challenged by lack of normal reference intervals and due to large intra‐ and inter‐patient variability reported in fractional excretion [10]. Fractional excretion measurement has not been shown to be of additional value over monitoring BUN and creatinine trends in the veterinary ICU [10,26].
The renal system
Introduction
Prerenal
Renal
Postrenal
Reduced effective circulating volume:
Reduced renal blood flow:
Infections:
Glomerular disease:
Renal neoplasia:
Pigment nephropathies:
Renal trauma:
Renal tubular disease/interstitial nephritis
Toxins
Environmental toxins:
Pharmaceuticals:
Diagnostic agents:
Supplements or dietary contaminants:
Renal tubular obstruction:
Ureteral obstruction:
Urethral obstruction:
Functional urinary retention:
Urinary tract leakage:
Initial assessment
History
Physical examination
Point of care testing
Initial clinicopathological testing
Diagnostic imaging
Radiographic studies
Contrast study
Indications
Patient preparation
Procedure
Notes
Excretory urogram
Subjective evaluation of GFR
Determination of renal size and position
Evaluation of size, and shape of renal pelvis
Evaluation for ureteral patency
Evaluation for ectopic ureter
Evaluation for patency or leaks from the level of the bladder to the ureters and urinary bladder
12–24‐hour fast
Serial enemas as needed to clear the colon of fecal material
Place IV catheter for contrast injection
Sedation as needed to achieve radiographs
Iodinated contrast should not be administered to patients who are dehydrated
Allergic reactions to iodinated contrast are uncommon and anaphylactic reactions rare
Contrast‐induced vomiting is transient and treated with supportive care
Contrast‐induced renal failure is uncommon but use of nonionic contrast is advocated to minimize complications
IV fluid administration post contrast is recommended to maintain hydration and induce diuresis
Negative‐contrast cystogram or pneumocystogram
Identify size, shape, and location of urinary bladder
12–24‐hour fast
Serial enemas as needed to clear the colon of fecal material
Sedation as needed to achieve urinary catheter placement and radiographs
Sterile urinary catheter placement for contrast injection
Radiographs can be taken with urinary catheter in place or removed
The negative contrast used can be air or medical carbon dioxide
Vascular air embolism from diseased urinary bladder wall is a rare but possible complication
Use of carbon dioxide may reduce the risk of air embolism
Positive contrast cystourethrogram
Identify size, shape, and location of urinary bladder
Evaluate for urinary bladder or urethral diverticula
Evaluate for urinary bladder or urethral patency or leakage
Evaluate for filling defects for urolith detection or hematomas
Evaluate for some bladder mucosal filling defects such as polyps or masses
Same patient preparation as a negative‐contrast cystogram
Iodinated contrast can be diluted with sterile saline (1:2 or 1:5 depending on the original concentration of iodinated contrast agent) and still achieve good opacification of urinary bladder and urethra
Radiographs with the pelvic limbs of the patient moved to different positions (pulled cranially or caudally) may be needed for good evaluation of the entire urethra
Contrast agent can be removed from the urinary bladder post procedure or the patient can be allowed to void the contrast
Double‐contrast cystogram
Identify size, shape, and location of urinary bladder
Evaluate for urinary bladder diverticula
Evaluate for urinary bladder leakage
Evaluate for filling defects for urolith detection or hematomas
Increased resolution for detection of bladder mucosal filling defects such as polyps, masses, and thickenings compared to positive contrast cystogram
Same patient preparation as a negative‐contrast cystogram
Undiluted iodinated contrast provides a better study than diluted contrast
The negative contrast used can be air or medical carbon dioxide
Vascular air embolism from diseased urinary bladder wall is a rare but possible complication
Use of carbon dioxide may reduce the risk of air embolism
Ultrasound
Finding
Diagnostic imaging
Radiography
Loss of abdominal detail and free peritoneal fluid (S)
Abdominal extravasation of contrast in urinary contrast studies (D)
Ultrasound
Free peritoneal fluid (S)
Observation of fluid flow directly from a urinary tract (D)
Fluoroscopy
Free peritoneal fluid (S)
Abdominal extravasation of contrast in urinary contrast studies (D)
Computed tomography
Free peritoneal fluid pockets (S)
Abdominal extravasation of contrast in urinary contrast studies (D)
Paired fluid and serum testing
Creatinine
Peritoneal fluid creatinine ≥2× serum creatinine (D)
Peritoneal fluid creatinine > serum creatinine (S)
Potassium
Peritoneal fluid potassium ≥1.4× serum potassium (D)
Peritoneal fluid potassium > serum potassium (S)
Advanced imaging
Uroscopic imaging
Glomerular filtration rate
Requirements
Equation
Creatinine clearance in mL/kg/min = (Ucr × Vurine/Scr x T)/BWkg
Where: Ucr = urine creatinine concentration in mg/ml, Vurine = total volume of urine collected in mL, Scr = Serum creatinine concentration in mg/mL, T = time in minutes of urine collection, BWkg = body weight in kilograms
Reference interval
Interpretation notes
Urine biomarkers
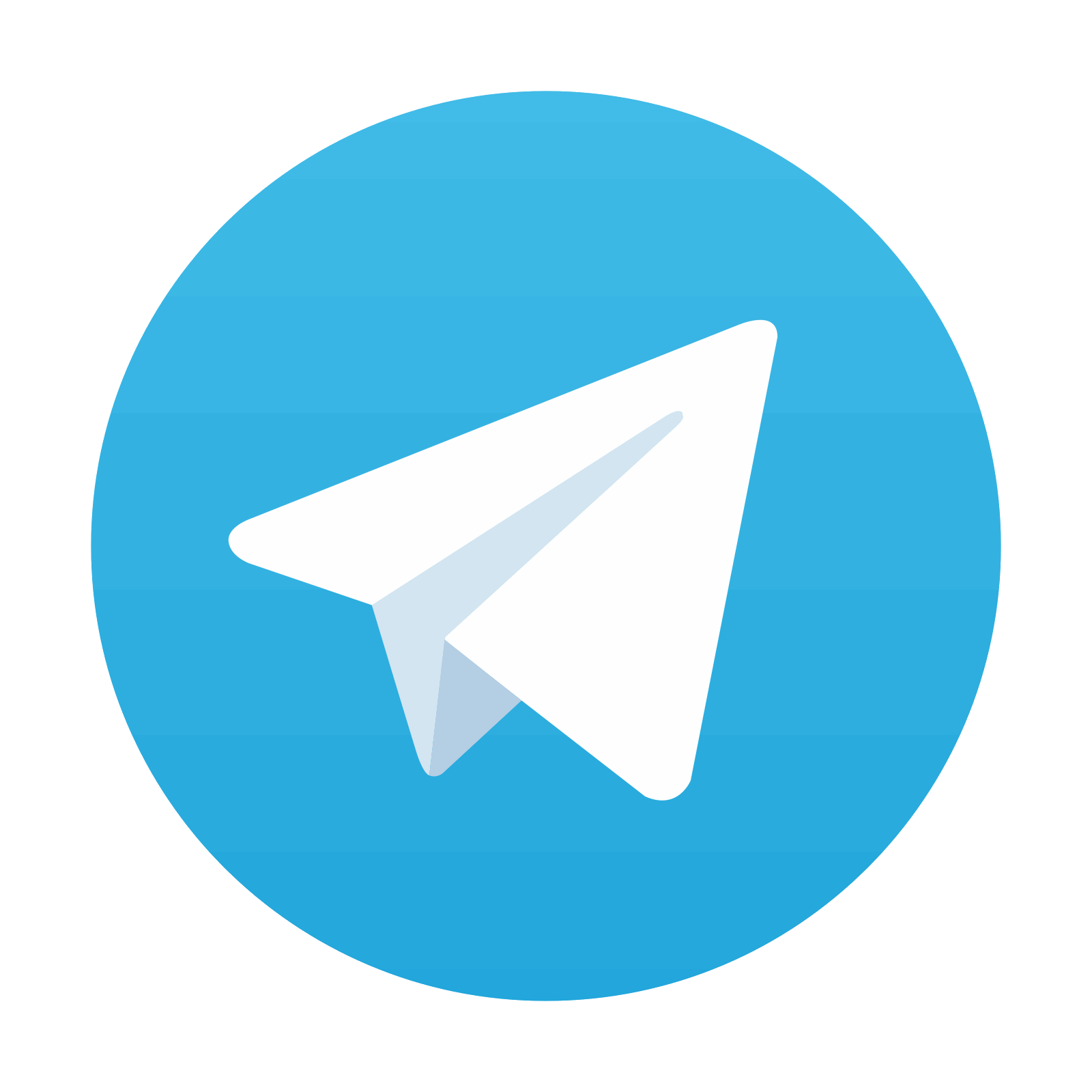
Stay updated, free articles. Join our Telegram channel

Full access? Get Clinical Tree
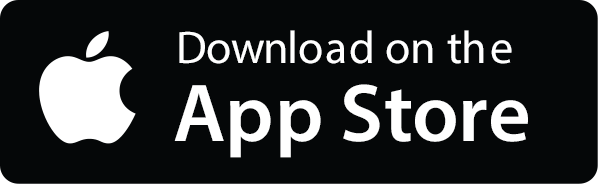
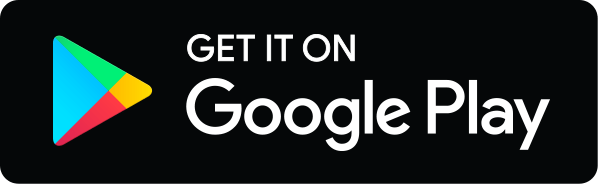