Nathan C. Nelson Department of Molecular Biomedical Sciences, College of Veterinary Medicine, North Carolina State University, Raleigh, NC, USA Indications for spinal imaging are numerous. Radiographs screen for occult spinal fractures in patients recently subjected to a traumatic event (hit by car, dog bite, etc.). Spinal imaging, particularly magnetic resonance imaging (MRI), is indicated in patients that are acutely paraplegic or display significant spinal pain. Patients with chronic limb neurologic deficits also warrant imaging, and MRI is the modality most likely to give a definitive diagnosis. Many patients with thoracic or hindlimb “lameness” in reality display nerve root signatures that localize to the spine, and rather than imaging the limbs, imaging tests should be directed toward the spine. Acquiring a thorough anamnesis and performing a careful orthopedic and neurologic examination are essential when determining the body part to image and imaging test (or tests) most likely to provide a diagnosis. As indications to image the spine are broad, availability, client cost, and the clinical question dictate the choice of modality. As an example, in a patient with a history of recent vehicular trauma displaying neurologic symptoms, radiographs are a quick and cost‐effective test to answer the question “Are there obvious fractures that could explain the clinical signs and would immediately alter the course of treatment?” If the answer is “no,” then the decision to either medically manage the patient or pursue advanced imaging with computed tomography (CT) or MRI must be made (keeping in mind the limitations of radiography in detecting subtle bone or soft tissue pathology). In comparison, a patient with more chronic neurologic symptoms localized to the nerve roots is less likely to benefit from spinal radiographs as soft tissue lesions would not be detected. CT is more widely available and less expensive than MRI, and displays greater sensitivity than radiography for many soft tissue lesions of the spine. For those reason, CT might be the best imaging test available for a client, even if MRI might ultimately be more the more sensitive test for some conditions. Despite the development of advanced cross‐sectional techniques, radiography remains the most common first‐line imaging test when evaluating patients with pain or neurologic symptoms localized to a spinal segment. Two orthogonal projections are acquired, albeit with caveats. Patients that have undergone trauma should initially have lateral radiographs performed, followed by ventrodorsal projections when there is confirmation that no gross spinal instability or fracture is present. In this situation, when orthogonal projections are required, cross‐table horizontal beam projections are a consideration as the patient does not need to be rolled into dorsal recumbency (as would otherwise be the case for ventrodorsal radiographs). Radiographs also allow careful stress radiography (with the vertebrae in flexion or extension), which is much more challenging with CT or MRI. Positional radiography can evaluate for spinal instability, such as with atlantoaxial instability. Radiographs readily identify the vertebral canal margins but the actual spinal cord is not seen due to effacement with surrounding soft tissues. Myelography involves injection of nonionic iodinated contrast medium into the subarachnoid space around the spinal cord, delineating its margins. Pathology that compresses or expands the spinal cord will be apparent. The subarachnoid space should remain a thin “column” that parallels the margin of the spinal cord. Spinal cord compression is recognized by distortion or narrowing of the subarachnoid space, with different patterns depending on whether the pathology is outside the dura mater (extradural pathology), within the spinal cord (intramedullary pathology), or between the dura mater and spinal cord (intradural‐extramedullary) (Figure 12.1). FIGURE 12.1 Relevant spinal anatomy, including dura mater, arachnoid membrane, and pia mater (A). Lesions in this area arise from the spinal cord (intramedullary), outside the dura mater (extradural) or within the dura but outside the spinal cord (intradural extramedullary) (B). Imaging tests attempt to determine whether pathology is located within one of these three areas, as that ultimately determines the most likely differential diagnosis present. Computed tomography provides better three‐dimensional anatomy of the spine and better soft tissue contrast than radiography, allowing earlier diagnosis of many spinal diseases compared to radiography. CT has advantages over MRI in that it is more accessible, cheaper, and faster. A typical CT examination of the spine lasts approximately 10 minutes from start to finish whereas an MRI examination is typically a minimum of 30 minutes. Unlike MRI, CT cannot distinguish the spinal cord as separate from the surrounding cerebrospinal fluid (CSF) or meninges. While it does allow visualization of the epidural space and other spinal structures, the soft tissue detail is inferior to MRI. CT may be combined with myelography, allowing better diagnosis by direct visualization of the spinal cord, more similar to MRI, but still provides limited internal detail of the spinal cord. Magnetic resonance imaging is the gold standard for imaging the spinal cord and paraspinal soft tissues. By separating the signal of CSF, spinal tissue, and epidural fat, spinal pathology is directly imaged without the need for intrathecal contrast agent. The downsides of MRI are the longer acquisition time, requirement of anesthesia, and greater expense of an examination. Typical MRI protocols include T2‐weighted and T1‐weighted images, pre‐ and post‐IV contrast. IV contrast is not necessary for all spinal examinations, particularly those where intervertebral disc extrusion is the most likely clinical diagnosis based on presentation and history. Withholding IV contrast saves time (as postcontrast images are unnecessary) and expense if the diagnosis is made on precontrast images. Numerous congenital anomalies affect osseous and soft tissue structures of the spine. Some of these have no clinical effect on the patient and do not result in clinical signs. Others present when the patient is young, as the malformation may result in early spinal cord compression. Some may not initially affect the patient, but may predispose to instability and present with clinical signs when older and instability becomes more pronounced. Atlantoaxial instability is a common disorder in small‐ and toy‐breed dogs. The etiology is complex, but typically involves both soft tissue and orthopedic malformations. Clinical signs are chronic and insidious, affecting all four limbs, though sometimes an acute exacerbation may be present. Though atlantoaxial subluxation may occur due to trauma, it typically is due to incomplete development of the soft tissues and osseous structures in this region. Specifically, ligaments that stabilize this joint are incompletely developed. Moreover, the dens may be shortened and hypoplastic, further leading to instability (Figure 12.2). The result is increased mobility at this joint, resulting in soft tissue inflammation, hypertrophy, and eventual spinal cord compression. FIGURE 12.2 Lateral (A) and VD (B) projections of a dog with dens hypoplasia (arrow) and C1–2 subluxation. Note the increased distance between the lamina of C1 (arrow) and spinous process of C2 (closed arrowhead). C2 is displaced dorsally relative to C1 and the dens is blunted (open arrowhead). Classically, diagnosis of atlantoaxial instability was achieved through a standard lateral radiograph performed in neutral position compared to a radiograph acquired during gentle neck flexion. Neck flexion results in separation of the lamina of C1 and the spinous process of C2, with dorsal displacement of C2 relative to C1, a motion prevented in normal dogs by the strong, thick atlantoaxial dorsal ligament. On the lateral projection, the normal alignment of C2 relative to C1 is altered, so that the angle of flexion between these vertebrae increases. Risk of spinal flexion in a dog with atlantoaxial instability is obvious, as flexion in a dog with instability can result in significant spinal cord compression as C2 subluxates dorsally and a worsening of clinical signs or even death. In recent years, other lesions associated with this instability have been recognized with the increased use of CT and MRI imaging. Specifically, atlantoaxial instability may be seen in concert with atlantooccipital overlapping, where the dorsal lamina of C1 protrudes into the caudal fossa of the skull and can result in cerebellar compression (Figure 12.3). This is only possible if there is concurrent malformation and incomplete ossification of the foramen magnum, resulting in a keyhole shape that allows C1 to protrude into the skull (often termed occipital dysplasia). This is difficult to recognize radiographically and requires either CT or MRI to definitively diagnose. FIGURE 12.3 Sagittal T1‐weighted MR (A) and sagittal CT (B) images of a dog with dens hypoplasia, C1–2 subluxation, and cranial displacement of C1 into the caudal fossa. The dens is blunted (arrow). The C1 lamina (closed arrowhead) is displaced cranially, being ventral to the dorsal margin of the foramen magnum (open arrowhead). The spinal cord is severely compressed at this level, but this is only appreciated on the MRI image as the spinal cord is not distinctly seen on the CT. Dens hypoplasia is commonly present in dogs with atlantoaxial instability (Figures 12.2 and 12.4). This is difficult to identify on a straight lateral projection of the spine as the large superimposed wings of C1 overlap the dens of C2, making identification of the dens difficult in all dogs. Slight, intentional obliquity of the head rotates the wings of C1 in a way that the dens may be appreciated (see Chapter 6). The ventrodorsal projection also highlights the dens and is another way to assess potential hypoplasia, though the risks of positioning a dog with suspected subluxation should be weighed against the information provided by a VD projection. Moreover, some dogs that are clinically normal may have undeveloped dens, so identification of this anomaly alone cannot diagnose instability without the dynamic positioning. FIGURE 12.4 Sagittal T2‐weighted MRI images of the dens in a normal dog (A) compared to a dog with dens hypoplasia (B). The dens in the normal dog (arrow) is much larger than the small one in the affected dog (arrowhead). There is also subluxation of C1–2 and spinal cord compression (arrowhead). Both MRI and CT are able to recognize morphologic abnormalities of C2 and C1, and can help confirm spinal cord compression, but dynamic imaging of the spine to confirm atlantoaxial subluxation is typically still reserved to radiography given the dangers with prolonged flexion required by MRI and CT to diagnose this instability. Transitional vertebrae have characteristics of two different vertebral types, and may be symmetric or asymmetric between their left and right halves. These anomalies typically are of the vertebral arch rather than the vertebral body. For instance, a thoracolumbar vertebral segment will have one lateral half that appears to be a thoracic vertebral body with a small adjacent rib, whereas the other lateral half will appear similar to a lumbar segment and have a broad transverse process (Figure 12.5). The most caudal ribs may be hypoplastic or aplastic bilaterally. Similarly, a lumbosacral transitional segment may have one lumbarized half and one sacralized half or both may be lumbarized. This may be seen involving only one vertebral segment (Figure 12.6) or multiple segments (Figure 12.7). FIGURE 12.5 Lateral (A) and VD (B) projections of a dog with congenital malformations at the thoracolumbar junction. The left 13th rib is aplastic and instead the left side of T13 appears similar to lumbar vertebrae (arrow). Multiple hemivertebrae are present which result in kyphosis (closed arrowhead). T10 is a butterfly vertebrae, as seen on the VD projection (open arrowhead). FIGURE 12.6 Lateral (A) and VD (B) projections of a lumbosacral transitional segment. S1 is separate from the other two sacral segments (arrow) and has two small lumbar‐like transverse processes (arrowheads). FIGURE 12.7 Lateral (A) and VD (B) projections of the lumbar spine in a dog with congenital malformations. The right 13th rib is hypoplastic with only a small remnant present (arrow), and the left 13th rib is broad and lumbarized (open arrowhead). L7 has broad transverse processes (closed arrowheads). Three fused sacral segments are present, best appreciated on the lateral projection. Transitional segments are most common at the thoracolumbar and lumbosacral junctions, but cervicothoracic transitional segments do occur, most commonly characterized by small vestigial ribs on C7. Those at the cervicothoracic and thoracolumbar junctions are typically incidental and do not result in clinical signs, though thoracolumbar transitional segments may complicate vertebral counting prior to spinal surgery and cause crowding of regional anatomy (Figure 12.8). Transitional segments at the lumbosacral junction increase the risk of intervertebral disc degeneration and instability, which can result in nerve impingement and cauda equina syndrome (Figure 12.9). Lumbosacral transitional segments may also affect normal hip development [1, 2]. Sacral congenital abnormalities are expected in some breeds (such as English bulldogs) and typically do not result in clinical signs (Figure 12.10). FIGURE 12.8 Lateral (A) and VD (B) projections of a dog with congenital malformations in the midthoracic region. T5–7 hemivertebrae have a wedge‐shaped vertebral body (arrowheads) and result in kyphosis. There is crowding of the ribs on the VD projection (arrows). FIGURE 12.9 Lateral (A) and VD (B) projections of a dog with a transitional first sacral segment. On the lateral projection, the first sacral segment (arrow) is unfused to the other two, and has an angulation between the lumbar and other sacral segments. On the VD, this vertebrae has short left and right margins which appear similar to lumbar transverse processes (arrowheads). This patient had cauda equina syndrome. FIGURE 12.10 Lateral projection of a bulldog with congenital malformations of the sacrum and caudal vertebrae, typical of the breed. The sacrum has a convex shape on the lateral projection, and multiple malformed caudal vertebrae are seen (arrow). The L6–7 disc is mineralized in situ (arrowhead). Hemivertebrae are congenital vertebral body malformations that represent a defect that occurred during vertebral body formation [3]. They are the most common malformation seen in the spine, and are most common in brachycephalic breeds such as the pug and English bulldog. They are most common in the midthoracic region, but occur as far caudal as the thoracolumbar junction, and are rare in the cervical or lumbar area (Figure 12.5). They may result in deviation of the long axis of the spine, directing it dorsally (kyphosis), ventrally (lordosis), or to the side (scoliosis). This degree of deviation is typically mild, but hemivertebrae result in vertebral canal stenosis and spinal cord compression if severe (Figure 12.11). FIGURE 12.11 Lateral radiograph (A), sagittal T2W image (B) and transverse T2W image (C) of a dog with a T7 hemivertebrae (arrow) resulting in kyphosis. There is spinal cord compression (arrowheads) as seen on the MRI images due to this malformation and presumably intervertebral disc herniation. Though most dogs with hemivertebrae are neurologically normal, kyphosis in particular is a risk factor for development of clinical signs from spinal cord compression [3, 4]. Classification schemes for the different shapes of hemivertebrae are based on which portions of the vertebral body are incompletely formed and to what degree [3, 5]. In general, hemivertebrae have a wedge type of shape, with some degree of ventral and/or lateral vertebral body hypoplasia or aplasia [3, 5]. Hemivertebrae are not always wedge shaped, however, and median aplasia, where there is incomplete or absence of the central region of the vertebrae, results in a “butterfly vertebrae” recognized by the resemblance to a butterfly when evaluated on the ventrodorsal projection (Figure 12.5). In some dogs, there may be symmetric and incomplete development of a vertebral body (termed symmetric hypoplasia), resulting in a diffusely shortened vertebral body but this does not cause any spinal malalignment. Block vertebrae are congenital defects in segmentation, where adjacent vertebral developmental bodies do not separate into two different vertebrae. The result is one long vertebral segment with adjacent fusion of the vertebral bodies and possibly the vertebral arches (Figure 12.12). These are usually not clinically relevant and do not cause symptoms, but can predispose to intervertebral disc degeneration (Figure 12.13) and herniation (Figure 12.14) in the adjacent discs due to increased loading from a fulcrum effect [6]. FIGURE 12.12 Lateral radiograph of a dog with a C3–4 block vertebrae (arrow). The dog had no clinical symptoms. FIGURE 12.13 Lateral radiograph (A) and sagittal T2‐weighted MRI (B) of a dog with C5–6 block vertebrae (arrow) and disc compression of the spinal cord at C4–5 (arrowhead). The spinal cord has an hour‐glass shape and increased T2 signal consistent with atrophy and gliosis from chronic compression. FIGURE 12.14 Sagittal T2W image (A) and sagittal T1W image (B) of a dog with C2–3 and C4–5 block vertebrae (arrows). The spinal cord is not significantly compressed. There is also concavity of the caudal C3 endplate, consistent with disc herniation into the vertebrae (arrowhead) sometimes termed a “Schmorl’s node.” Incomplete development of the caudal articular processes of the vertebrae at the thoracolumbar junction has been described in brachycephalic dogs. These articular processes may be either hypoplastic or completely aplastic, resulting in decreased vertebral column stability (Figure 12.15). Pugs are particularly affected. This malformation is associated with progressive neurologic symptoms from chronic cord disease from chronic instability. This may be recognized on survey radiographs by incompletely formed or absent articular processes, but is most easily recognized when cross‐sectional imaging is performed in these patients (Figure 12.16) [7]. FIGURE 12.15 3D image of a pug with facet hypoplasia (A) and a pug without facet hypoplasia (B). In the affected dog, multiple caudal articular facts are absent or hypoplastic (open arrowheads) when compared to the nonaffected dog where the caudal articular facets are normal (closed arrowheads). Note that in (A) there is hypoplasia of the right 13th rib (arrow) and in (B) there is a lumbarized transitional right 13th rib. FIGURE 12.16 Transverse T2W image (A) and transverse CT image (B) of a dog with facet hypoplasia, compared to CT images of a normal dog (C). These are the same dogs as in Figure 12.15. On MRI images, there is a herniated disc causing spinal cord compression (arrowhead) at T12–13. A transverse process is unilaterally absent (closed arrowhead) in the affected dog (closed arrowhead) but left and right transverse processes are present in the normal dog (open arrowheads). Spina bifida is a defect in the normal embryologic development of the neural tube, characterized by failure of the vertebral arches/lamina to close over the spinal cord [8]. This is recognized by a duplication of the spinous process to the left/right sides of the midline on the ventrodorsal radiograph or absence of normally formed spinous processes (Figure 12.17). Typically, this malformation is not associated with neurologic defects and the underlying meninges and spinal cord are presumed normal. If instead, the meninges protrude through the open vertebral arch, the defect is referred to as meningocele, and if the meninges and neural tissue extend into the opening, it is referred to as meningomyelocele [8]. Radiographs cannot determine the degree of meningeal/neural involvement, which usually relies on MRI, though CT with a myelogram would accurately assess the degree of neural involvement (Figure 12.18). FIGURE 12.17 VD radiograph of a dog with spina bifida. There is incomplete formation of the L6/7 lamina with lack of distinct spinous process (arrows). FIGURE 12.18 Transverse (A) and sagittal (B) T2W MRI images of a dog with spina bifida and a meningomyelocele. This is the same dog as Figure 12.17. The subarachnoid space protrudes through this defect (arrowheads). Note that the nerve roots of the cauda equina extend into this defect (arrow). Osteosclerosis is defined by a diffuse increase in opacity of long bone and vertebral medullary cavities (Figure 12.19). This radiographic appearance is occasionally seen in young or older patients, and may be attributed to multiple disease processes, though the cause is not always identified. Osteopetrosis is a rarely reported inherited bone disease caused by abnormal osteoclast function, resulting in osteosclerosis in young patients. Osteosclerosis has been described in cats infected by feline leukemia virus and also described as a paraneoplastic change [9]. FIGURE 12.19 Lateral (A) and VD (B) radiographs of a cat with generalized osteosclerosis. There is diffuse increased opacity of the medullary bone, including the ribs and humeri. This rare congenital disorder in dogs and cats is caused by an abnormality somewhere along the hypothalamic‐pituitary‐thyroid axis, resulting in decreased production of thyroid hormones. Most commonly, it is due to dysgenesis of the thyroid gland [10, 11]. As thyroid hormone is necessary for normal bone development, all bones are equally affected, with animals displaying disproportionate dwarfism with delayed epiphyseal maturation and epiphyseal dysgenesis [11]. In the spine, the vertebrae will have a shortened appearance and are often decreased in opacity (Figure 12.20). There may be secondary degenerative changes to the spinal articular joints. FIGURE 12.20 Lateral cervical (A) and lumbar (B) radiographs of a dog affected by congenital hypothyroidism with cervical (C) and lumbar (D) radiographs from a normal age‐matched dog. There is generalized decreased bone opacity and small epiphyses of the affected dog when compared to the normal dog. Osteogenesis imperfecta is a well‐documented but rare heritable bone disease that results in diffuse skeletal maldevelopment, sometimes referred to as “brittle bone disease” [12, 13]. The cause is a defect in the genes that encode the precursor molecules to collagen type 1, which makes up 90% of the organic substance of bone. Defects in this matrix reduce overall bone mineralization. These weakened bones are predisposed to folding fractures and are frequently malformed. Patients will commonly appear stunted. Radiographically, there is diffuse decrease in opacity of the vertebrae, and endplates and cortical bone will appear thin (Figure 12.21). Fractures and malformation of the spine are typical. Because this is a diffuse disease, similar changes are expected in the long bones. FIGURE 12.21 Lateral radiograph of a dog with osteogenesis imperfecta. There is decreased bony opacity diffusely. Multiple rib fractures (arrow) are present and spinal deformations (short vertebrae, lordosis) from previous folding fractures. Patients fed a diet with inappropriate levels of calcium and vitamin D are at risk of nutritional hyperparathyroidism. The resultant mobilization of calcium from osseous structures results in vertebrae which are more lucent than expected (Figure 12.22). The overall appearance is similar to osteogenesis imperfecta, but the conditions are readily differentiated when a complete diet history is collected. FIGURE 12.22 Lateral radiograph of a cat with nutritional hyperparathyroidism. Notice that all bones are decreased in opacity relative to adjacent soft tissues, resulting in poor osseous contrast. Discospondylitis is infection (usually bacterial) of the intervertebral disc. The infection typically spreads to the disc through a hematogenous route, though rarely infections may be fungal in etiology and caused by local infections (such as those caused by epidural infections or migrating grass awns; Figure 12.23) [14]. The infection starts in the cartilaginous endplate of the vertebral body, and is recognized radiographically when lysis of the osseous endplate develops [14]. The bacterial source is most commonly in the urinary tract or prostrate, as bacterial infections in this area may enter the bloodstream and lodge in the vertebrae via venous drainage of the caudal abdomen that flows through the venous sinuses. FIGURE 12.23 Lateral cervical radiograph showing C5–6 discospondylitis (arrow). There is ventral bony proliferation and widening of the disc space due to irregular endplate lysis. Radiography is the most common screening method for discospondylitis. The osseous endplates demonstrate permeative or moth‐eaten lysis causing an irregular margin (Figure 12.24). The adjacent vertebral body responds by bony deposition and a region of increased opacity. With time, the intervertebral disc space will collapse (Figure 12.25). Bony proliferation occurs at the margin of the vertebral bodies, with an appearance similar to spondylosis deformans. In juvenile dogs less than 6 months of age, intervertebral disc narrowing may occur before endplate lysis [15]. FIGURE 12.24 Lateral radiograph of a dog with L6–7 discospondylitis. Disc collapse has occurred with narrowing of the disc space. The endplates of L6–7 are sclerotic but lysis is subtle (arrowhead). There is ventral soft tissue swelling (arrow).
CHAPTER 12
Imaging of the Spine
Introduction
Modalities for Imaging the Spine
Radiography
Myelography
Computed Tomography
Magnetic Resonance Imaging
Congenital Vertebral Disorders
Atlantoaxial Subluxation
Transitional Vertebrae
Hemivertebrae
Block Vertebra
Facet Dysplasia
Spina Bifida
Uncommon, Diffuse Bone Diseases
Osteosclerosis
Congenital Hypothyroidism
Osteogenesis Imperfecta
Nutritional Secondary Hyperparathyroidism
Intervertebral Disc Diseases
Discospondylitis
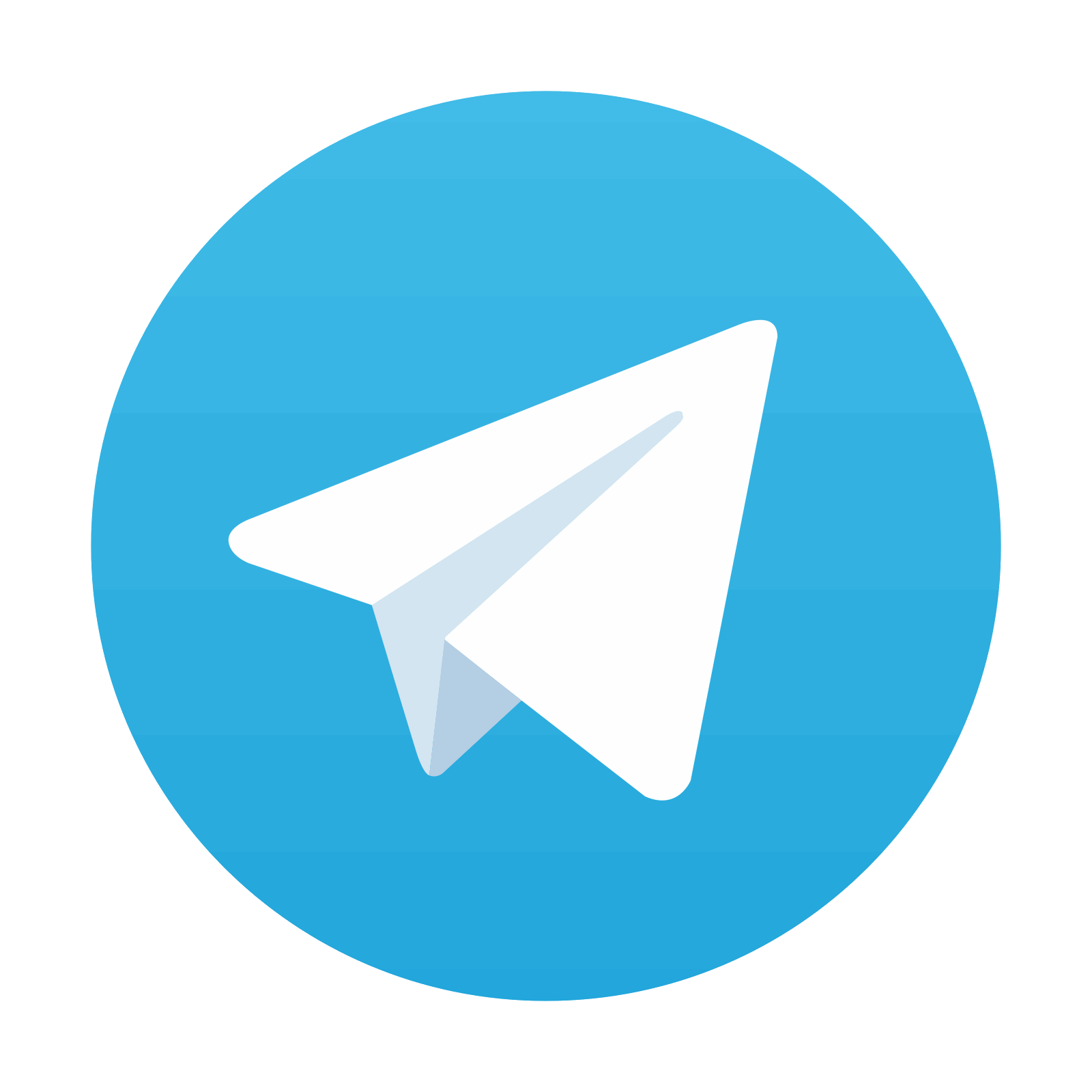
Stay updated, free articles. Join our Telegram channel

Full access? Get Clinical Tree
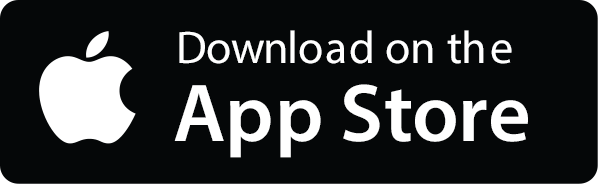
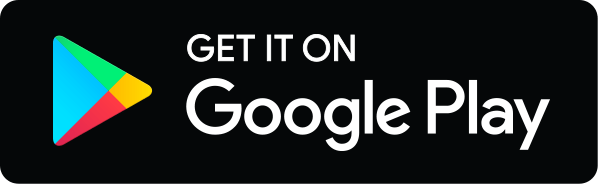