CHAPTER 11 Plant Chemistry in Veterinary Medicine: Medicinal Constituents and Their Mechanisms of Action
Botanical medicines owe their activity to their multitude of constituents. These constituents are generally broken up into the categories of primary and secondary, that is, those essential for life and those with nonessential functions, respectively. This chapter reviews the major categories of plant compounds and their mechanisms of action, when they are known. Notes about potential differences in the effects of various plant constituents on different animals are stated, to the extent that they are known.
Secondary metabolites clearly provide most of the therapeutic activity of medicinal plants and fungi (Yarnell, 2003). Concurrent evolution of animals, plants, and fungi helps explain why this is so. Animals that are able to make use of plant secondary metabolites would be better suited to survive and spread the genes that allow for the benefits gained. This can be viewed as creating a genetic arms race in which plants and fungi evolve secondary metabolites to interfere with animal predators that are successfully exploiting plant compounds. It can also be viewed cooperatively, for animals can be hijacked by plants or fungi in the process, as occurs when animals become seed or pollen dispersers. Regardless, knowledge of secondary metabolites is critical to an understanding and the effective application of modern herbal medicines.
POLYSACCHARIDES
Although most complex carbohydrates are technically water insoluble, they do form gels to varying degrees when mixed with water. Basically, water adsorbs into the hydrophilic pockets within and among the polysaccharide chains, leading to partial weak association of chains via hydrogen bonding or coordination. These gels are generally reversible with addition of heat, although warm water is most effective in the creation of most gels. Some gel formation involves the formation of full covalent bonds and may be irreversible. These gels ultimately end up as insoluble precipitates.
The molecular mechanisms of action of polysaccharides and polyuronides have been most extensively studied as the dietary fiber portion of foods. Dietary fiber, likely through short-chain fatty acid breakdown products produced by gut microbes, decreases hepatic synthesis of fatty acids and thus reduces triglyceridemia (Delzenne, 1998). Fiber also increases bile acid turnover and modifies its synthesis, thus lowering systemic cholesterolemia (Levrat, 1994). Only soluble fibers have these effects consistently (Kay, 1980). Hypolipidemic effects generally require several weeks of treatment, as gene expression, microbial flora alterations, and other changes take time to occur after exposure to high-fiber diets (Delzenne, 1999).
Dietary fiber induces satiety and slow gastric emptying, apparently according to the viscosity of the material. In a normal nonruminant gut, fiber tends to reduce transit time, with variable strength depending on the type of fiber involved. When diarrhea is present, however, dietary fiber actually binds the stool and increases transit time. Fiber has also been shown in many studies to decrease formation of colon cancer in animals exposed to carcinogens, although much work remains to be done to elucidate the degree to which this effect is clinically relevant (Alabaster, 1993). The anticarcinogenic effects and many other properties of fiber may in part relate to its multifactorial effects on the gut flora, although at present, the exact effects of various types and amounts of fiber to be used for different durations of time have not been completely clarified (Woods, 1993). Fiber also reduces paracellular gut permeability in rodents (Mariadason, 1999).
Fiber in the gut delays absorption of glucose, which, in turn, reduces insulin responses (Kritchevsky, 1988). High-fiber diets have been extensively studied for their antidiabetic properties.
Gel-forming complex carbohydrates also act as demulcents or emollients. A demulcent is hydrating to the skin and moderately anti-inflammatory; it protects the gastric and esophageal epithelium from acid or other erosive materials. No research is available on the exact mechanisms involved in demulcent effects, other than the obvious mechanical barrier functions that they perform on tissue surfaces. The demulcent property is exploited clinically primarily to treat inflammatory conditions of the skin and digestive system. The immediate soothing effect of application of infusions of demulcent herbs has been demonstrated in clinical trials on humans with pharyngitis (Brinckmann, 2003).
Demulcents are believed to stimulate mucus production in the respiratory and urinary tracts via nerve reflexes, although this hypothesis has not been systematically assessed. One study of Althaea officinalis (marshmallow) root extract and polysaccharide isolate in cats showed that, at 100 and 50 mg/kg body weight doses, respectively, they acted as significantly more potent antitussives than the drug prenoxdiazine (Nosal’ova, 1992). Because complex polysaccharides are very unlikely to circulate in any quantity, it is difficult to imagine how they could act as antitussives, unless this occurs through some indirect mechanism.
Complex polysaccharides administered orally have repeatedly been shown to have immunostimulating effects. For example, polysaccharides from the root of Rehmannia glutinosa, an Asian medicinal herb, have been shown to inhibit complement (Tomoda, 1994). The polysaccharides of the root of Glycyrrhiza uralensis (gan cao), a close relative of licorice, and of the seed of Plantago asiatica (Asian plantain) have been shown to stimulate phagocytosis (Tomoda, 1990; Tomoda, 1991). Sulfated galactans from thallus of Chondrus ocellatus, a Chinese seaweed related to Chondrus crispus (Irish “moss,” also actually a seaweed), have shown multiple complex effects on various components of the immune system (Zhou, 2004). Detailed molecular mechanisms for the immune effects of polysaccharides are largely unknown, although in the case of Echinacea purpurea leaf and flower polysaccharides, evidence suggests that its immune-stimulating effects are due to changes in levels of various interleukins (Parnham, 1999). Evidence for the proposed immunomodulating effects of polysaccharides, that is, their ability to both stimulate and suppress various immune cells depending on the milieu, was not located (Box 11-1).
BOX 11-1 Actions of Gel-Forming Polysaccharides
Polysaccharides can delay absorption of some drugs, such as digoxin, which is enterohepatically resorbed (Brown, 1978). Monitoring the blood levels of drugs in animals fed large amounts of polysaccharides may be necessary to ensure therapeutic effects in some cases (Box 11-2).
GLYCOSIDES
The glycosidic linkage is relatively fragile. Several enzymes exist that can break the link, including beta-glucosidase and beta-galactosidase. It is unclear whether these enzymes exist in the gastrointestinal lumen of most animals but they almost always occur in their livers. Gut flora also typically possess these enzymes. Heat can break the bonds. According to one study of glycoside breakdown in leaves of Senna alexandrina (senna), 30° C (86° F) and 60% relative humidity and 40° C (104° F) and 75% relative humidity were sufficient to cause significant glycoside loss compared with 25° C (77° F) and 60% relative humidity (Goppel, 2004). A methanol extract of senna showed much less breakdown except at even higher temperatures. Ultraviolet light and extremes of pH can also break some of these bonds. Because of the changes in pharmacokinetics and possibly pharmacodynamics of herbs with intact glycosides versus free aglycones, many botanical practitioners believe that it is important for herbs containing glycosides to undergo minimal processing.
The pharmacokinetics of glycosides are complex but central to an understanding of their importance. A simple model is that of a built-in delayed release system, in which the hydrophilic glycoside delivers a hydrophobic aglycone to the large intestines. Most intact glycosides generally pass through the stomach and small intestines unchanged. However, some glycosides are absorbed, apparently by the intestinal epithelial intestinal Na+/glucose monosaccharide cotransporter, SGLT-1 (Wagner, 2003).
Once in the colon, the glycosidic bond is hydrolyzed by enzymes possessed by the gut flora. Some aglycones released by this process may also undergo enzymatic modification by gut bacteria. Aglycones are then absorbed via the large intestine, undergo first-pass metabolism in the liver, and circulate to varying degrees within the body. This process has been extensively studied, for example, with the glycosides in Panax ginseng (Asian ginseng) roots, known as ginsenosides (Hasegawa, 2000). Ginsenoside aglycones released by the action of gut flora have been shown to be more potent antineoplastic compounds compared with intravenously injected intact ginseno-sides in mice (Wakabayashi, 1997). Some ginsenosides are esterified to fatty acids in the liver and may have prolonged half-lives as a result (Hasegawa, 2004).
Cardiac Glycosides
Cardiac glycosides with long half-lives (4 to 6 days in the case of digitoxin) accumulate in the body. This greatly increases the danger of toxicity. Those glycosides that are rapidly cleared, such as those found in Convallaria majalis (lily of the valley), do not accumulate and are of mild potency. Those that accumulate, such as those found in Digitalis purpurea (foxglove), are of strong potency and must be used cautiously. Major common adverse effects caused by overdose include anorexia, nausea, vomiting, arrhythmia, confusion, headache, depression, green or yellow tinting of the vision, blurred vision, and worsening CHF. The toxic and therapeutic doses of Digitalis and potent, isolated cardiac glycosides can be very close, and sometimes toxicity is noted before therapeutic benefit. These problems are rarely noted with milder herbs (Box 11-3). Because even mild potassium depletion can greatly increase the toxicity of all cardiac glycosides, it is critical for the practitioner to avoid combining these agents or herbs that contain them with potassium-depleting medications (Chung, 1969). Of particular concern are non–potassium-sparing diuretics, corticosteroids, and Glycyrrhiza glabra (licorice). Antibiotics may also unpredictably alter the effects of cardiac glycosides by changing the gut flora, thus altering enterohepatic recirculation. Any change in metabolism, such as fever, kidney failure, or intervening hyperthyroidism, can also dramatically alter the pharmacokinetics and thus the pharmacodynamics of these compounds.
Anthraquinone Glycosides
Anthraquinone glycosides are generally orange, red, or brown-red compounds found in fairly limited distribution within the plant kingdom. Their solubility is similar to that of other glycosides with hydrophobic aglycones. Although some aglycones are absorbed within the small intestines, many of the intact glycosides make it to the colon before being hydrolyzed. In traditional Chinese medicine, this fact was exploited, although the rationale was explained differently. Herbs containing anthraquinone glycosides, particularly Rheum palmatum (rhubarb), were administered boiled if noncathartic properties were preferred because this would hydrolyze many of the anthraquinone glycosides. According to one review, boiling reduces the cathartic effects of rhubarb root 50% (Natori, 1981). Nonboiled forms of the herb were administered when catharsis was the desired outcome.
Some percentage of the anthraquinones are deposited in the colon wall, leaving dark brown or black patches referred to as pseudomelanosis coli. Most clinical research in humans confirms that this does not promote colorectal cancer (Yarnell, 2000). It is interesting to note that much research in fact shows that anthraquinones are antineoplastic. Emodin, found in rhubarb, is an apoptosis inducer (Lee, 2001).
Anthraquinones have other interesting properties. They are antifungal, inhibit excessive renal tubular cell proliferation, delay deterioration of patients in renal failure, modulate inflammation by partially inhibiting cyclooxygenase, and so forth (Agarwal, 2000; Guo, 1989; Sanada, 1996; Zheng, 1993). As far as is known, subcathartic doses or doses of noncathartic forms of herbs containing anthraquinone glycosides do not cause any serious adverse effects and will not cause pseudomelanosis coli. The aglycones can discolor the urine and feces red, brown, or black as they are excreted (Box 11-4).
Cyanogenic Glycosides
Cyanogenic glycosides have amino acid–derived aglycones that are mostly a safety concern in medicinal plants. The concerns about cyanogenic glycosides are twofold. First, these compounds interfere with iodine organification and thus can cause or promote goiter and hypothyroidism. However, this problem has been clearly documented to occur only in the setting of iodine deficiency or with massive overconsumption. Second, cyanogenic glycosides do spontaneously degrade to release potentially lethal hydrogen cyanide once the glycosidic linkage is hydrolyzed. However, unless massive and rapid intake of cyanogenic glycosides occurs, the cyanide is quickly and safely detoxified by hepatic thiosulfate sulfurtransferase. Smaller animals, juveniles, and herbivores that eat large amounts of Prunus spp leaves or white clover are potentially more susceptible. Other symptoms of acute cyanide exposure include headache, bronchial constriction, and weakness (Magnuson, 1997). Toxic effects, if any, of chronic, low-level exposure are unknown.
Evidence suggests that cyanogenic glycosides may have beneficial effects in animals that consume them. The most controversial evidence involves the cyanogenic glycosides from Prunus spp (cherry), particularly amygdalin (sold under the trade name Laetrile) and prunasin. These compounds do have anticarcinogenic activity in vitro according to recent research (Fukuda, 2003). Previous animal and human studies have, however, failed to show convincing clinical effectiveness of isolated injections of amygdalin (Hill, 1976; Moertel, 1982). Prunasin has also been shown to inhibit DNA polymerase in vitro (Mizushina, 1999).
GLUCOSINOLATES
Isothiocyanate aglycones such as phenethyl isothiocyanate (PEITC) and sulforaphane and glucobrassicins indole-3-carbinol (I3C) and diindolylmethane (DIM) have been widely studied because of their presence in broccoli and other commonly consumed Brassicaceae vegetables. These compounds have complex effects on cytochrome P450 (CYP) isoforms 1A1, 1A2, and 2B1 in various parts of the body, but ultimately, they tend to decrease hepatic formation of carcinogenic metabolites from a wide variety of environmental chemicals (Smith, 2000; Shertzer, 2000). One of the best studied properties of I3C in particular is its ability to induce the 2-hydroxylation catabolic pathway for estradiol, which deprives the 16-alpha and 4-hydroxylation pathways of substrate and appears to greatly reduce the negative effects of estradiol (Bradlow, 1995). This is because 2-hydroxylation metabolites are vastly weaker estrogens than 16-alpha- or 4-hydroxylation metabolites (Bradlow, 1995).
Glucosinolate aglycones are excreted by the kidneys after conjugation to glutathione by the action of glutathione-S-transferase (GST) or after conjugation to N-acetylcysteine. It has been shown that humans who have the GSTM-1 and GSTT-1 null genotypes do not excrete glucosinolate aglycones efficiently; thus, these stay in their bodies longer after ingestion. This correlates strongly with the anticancer effects of these compounds and helps explain why some people derive protection from cancer by eating Brassicaceae vegetables, while others do not (Lin, 1998; Spitz, 2000).
Glucosinolates can cause nausea and, in overdose, vomiting. This property is sometimes used in emergencies when no access to medical care is available, to induce vomiting through administration of large quantities of mustard powder. Other adverse effects resulting from internal use have not been documented. Because of their complex effects on CYP enzymes, their use with prescription drugs may cause relevant interactions. For example, it has been shown that reasonable doses of garlic reduce serum levels of protease inhibitors in healthy volunteers, consistent with CYP3A4 induction (Piscatelli, 2002).
FLAVONOIDS AND PROANTHOCYANIDINS
Flavonoids share a central three-ring structural motif and are all synthesized from cinnamic acid. Numerous subcategories of flavonoids exist, depending on the various functional groups present (Box 11-5). Note that the term bioflavonoid is redundant and thus should not be used. Proanthocyanidins are oligomers of flavonoids. Condensed tannins are large polymers of flavonoids. Thus, these three categories of constituents are closely related biosynthetically, and, at least in the case of flavonoids and proanthocyanidins, chemically (Table 11-1). Condensed tannins develop very distinct properties from these other two types of molecules and thus are discussed in a separate section.
Flavonoids and proanthocyanidins are almost universally antioxidant. They are believed to have this function in the plants or fungi in which they are found; thus, it is not surprising that they would do the same for anyone who consumes them. Many flavonoids and proanthocyanidins also generally tend to decrease capillary permeability and fragility, although at least one line of evidence suggests that the flavan-3-ol subgroup of flavonoids are the only ones that have this action, sometimes referred to as the “vitamin P [permeability]” effect (Roger, 1988). Lesions caused in blood vessels by vitamin C and flavonoid deficiencies in rodents are distinctive on a microscopic level (Casley-Smith, 1975).
Flavonoids, proanthocyanidins, and vitamin C have a complex and unclear relationship. Careful in vitro examination found that only dihydroquercetin, out of many flavonoids tested, could reduce oxidized vitamin C, contrary to the general belief that all flavonoids can do this (Bors, 1995). Another research group found that vitamins C and E were not synergistic antioxidants with various flavonoids in liposomes, but that their effects were merely additive (Vasiljeva, 2000). One in vitro study showed that some flavonoids, such as fisetin and quercetin, inhibited squamous cell carcinoma cells from growing only in the presence of ascorbic acid (Kandaswami, 1993). Much more research is clearly needed, but the strong link between flavonoids, proanthocyanidins, and vitamin C in the public mind is not solidly supported in the existing literature.
Other molecular actions of flavonoids and proanthocyanidins are extremely diverse, depending on the compound in question. For example, many flavonoids and proanthocyanidins are anti-inflammatory and antineoplastic. Numerous recent studies suggest that this is so because, at least in part, of the ability of these compounds to inhibit the NF-kappa-B signaling pathway that triggers inflammatory cascades (Moreira, 2004); they also inhibit mitogen-associated protein kinase (MAPK) and induce apoptosis, possibly through activation of c-Jun NH(2)-terminal kinase (JNK)–mediated caspase (Vayalil, 2004; Hou, 2004). Many flavonoids are also antiallergic. The aglycone of herperidin, hesperidin, released by action of the gut flora, inhibited immunoglobulin E (IgE)-induced histamine release as effectively as the drug azelastine in vitro (Lee, 2004).
Isoflavones, which are structurally closely related to flavonoids, also occur as glycosides. Although these compounds have multiple activities, much research has focused on their function as phytoestrogens. Note that other constituents, notably some lignans, can also be phytoestrogenic. This means that they act as weak estrogen receptor (ER)-β agonists (Morito, 2001). Most phytoestrogens also bind and agonize ER-α, but much more weakly than ER-β; however, exceptions do exist (Kuiper, 1998). Because phytoestrogens are 100 to 100,000 times weaker agonists than estradiol, they are functional estrogen antagonists when ingested by animals with normal levels of estradiol (Bickhoff, 1962; Shutt, 1972). This interaction is particularly important to consider because many in vitro studies report that phytoestrogens stimulate ER-positive breast cancer cells, unless estradiol is added to the system, in which case, inhibition of breast cancer cells has been noted (Schmitt, 2001). The clinical effects of phytoestrogens thus depend on endogenous estrogen status; they can be conceptualized as balancing estrogen levels.
Sufficiently high levels of phytoestrogens can clearly induce reproductive changes in ruminants that are consistent with estrus (Nwannenna, 1995). A genistein dose of 0.7 mg daily in mice has shown osteoprotective effects, and 5 mg daily was sufficient to cause uterine hypertrophy, clearly indicating that levels of exposure within the system can cause very different effects (Ishimi, 2000). Contrary to the level of information available, the isoflavones genistein and daidzein are present at much higher levels in Pueraria montana (kudzu) root and several beans other than Glycine max (soy) fruit (Kaufman, 1997).
One of the great advantages of flavonoids and proanthocyanidins is that they are essentially nontoxic. Their widespread presence in foods means that most animals are exposed to gram quantities of total flavonoids and proanthocyanidins on a daily basis with no obvious signs of problems. The concern about carcinogenicity of some flavonoids, particularly quercetin, have not played out in animal studies unless absurdly high doses are used and do not fit with human epidemiologic data suggesting that diets rich in flavonoid- and proanthocyanidin-containing foods are protective against cancer (Hertog, 1996; Hertog, 1994). Part of the safety lies in the relatively short half-lives of most compounds, which, for example, were found to be 2 to 3 hours in one human study of the anthocyanidin glycosides from Hibiscus sabdariffa (roselle) (Frank, 2005).
< div class='tao-gold-member'>
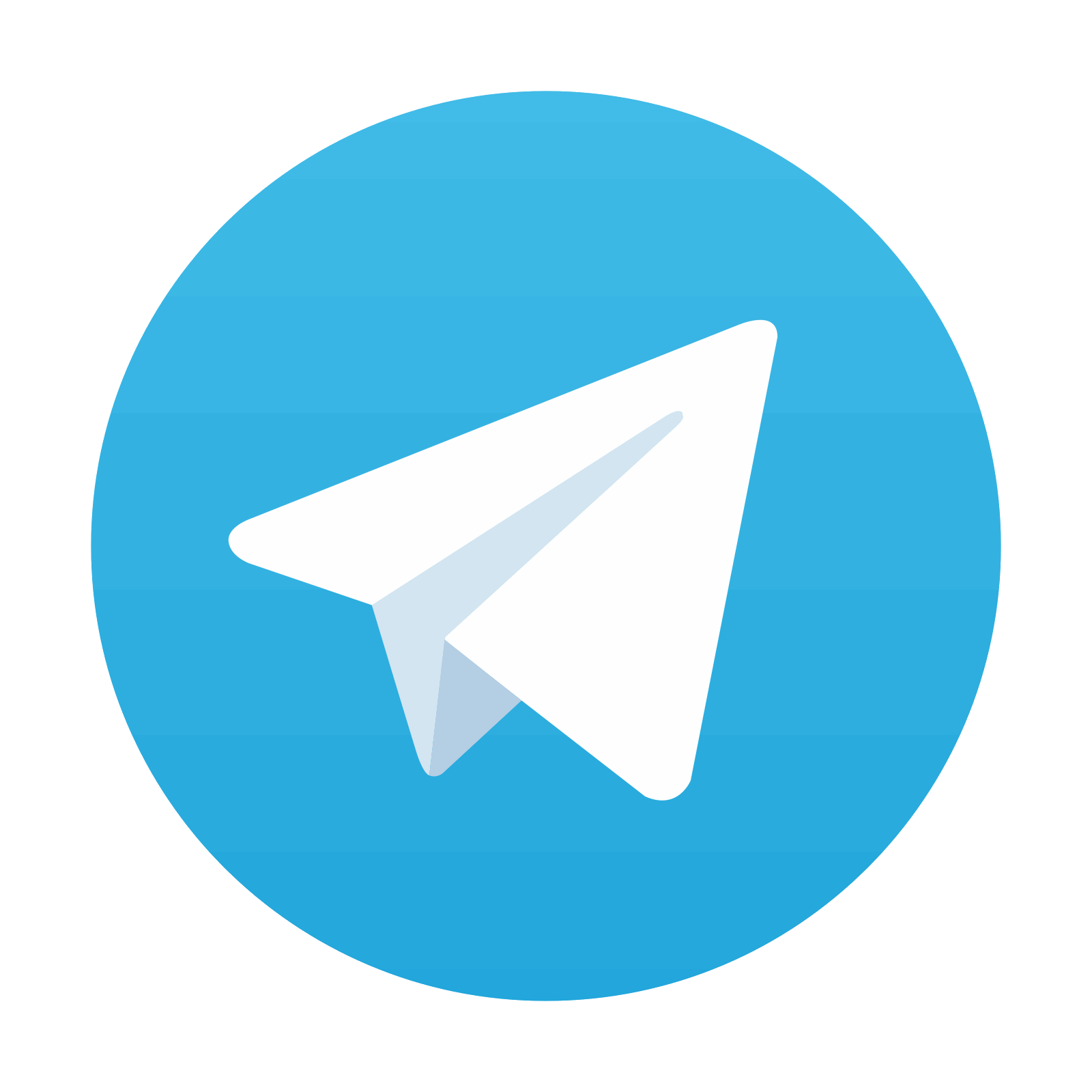
Stay updated, free articles. Join our Telegram channel

Full access? Get Clinical Tree
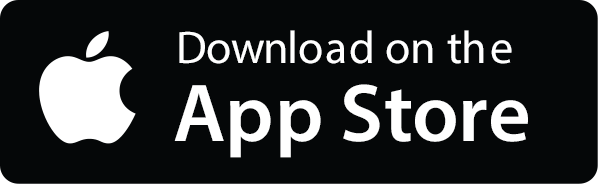
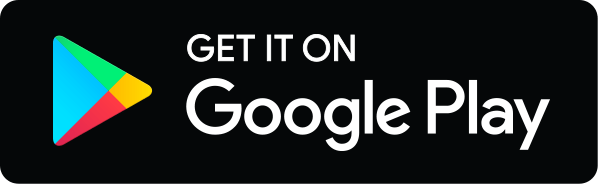