CHAPTER 11 Dennis E. Burkett Hope Veterinary Specialists, Malvern, Pennsylvania The primary function of the cardiovascular system is to provide oxygen and substrate to the tissues for energy production. The heart is the pump that controls the flow of blood and must continuously adjust to meet the demands of the body for oxygenated blood. This cardiac pump has a right and left side, each composed of an atrium resting above a heavily muscled ventricle (Figure 11.1). The left ventricular wall is normally significantly thicker than the right. Cup‐shaped valve leaflets (tricuspid on the right, mitral on the left) separate the atria from the ventricles. A thick muscular septum separates the right from the left ventricle. The right atrium receives blood from the systemic circulation and delivers it to the right ventricle. From here, the blood is pumped through the pulmonary artery into the pulmonary circulation for oxygenation and passive diffusion of carbon dioxide. The left atrium receives the oxygenated blood from the pulmonary veins and delivers it to the left ventricle. From here it is pumped into the systemic circulation through the aorta. Figure 11.1 Normal anatomy, pressures (systolic/diastolic/mean), and oxygen saturations (in circles) in the chambers of the heart, systemic circulation, and pulmonary circulation. Source: Kittleson MD, Kienle RD, eds. Normal clinical cardiovascular physiology. In: Small Animal Cardiovascular Medicine. St Louis: Mosby. 1998. Used with permission of Elsevier [1]. Ao, aorta; LA, left atrium; LV, left ventricle; PA, pulmonary artery; RA, right atrium; RV, right ventricle. This pumping action of the heart occurs in a cyclical fashion through myocardial contraction and relaxation. This is described as the cardiac cycle and is illustrated in Figure 11.2. The myocardial cells undergo electrical depolarization, triggering calcium movement within the cell, illustrated in Figure 11.3. The period of ventricular contraction is termed systole and the period of relaxation is called diastole. Systole and diastole are further divided into phases of cardiac activity. Although the atria also have periods of systole and diastole, the cardiac cycle focuses on ventricular contraction and relaxation [1,2]. Figure 11.2 Schematic drawings of left heart pressures, aortic flow, left ventricular volume, heart sounds, venous pressure, and electrocardiogram on the same time scale. Events within the cardiac cycle are labeled. Source: Kittleson MD, Kienle RD, eds. Normal clinical cardiovascular physiology. In: Small Animal Cardiovascular Medicine. St Louis: Mosby. 1998. Used with permission of Elsevier [1]. Figure 11.3 A schematic diagram of the movements of calcium during excitation‐contraction coupling in cardiac tissue. Excitation‐contraction coupling is the process whereby an action potential triggers a myocyte to contract. When a myocyte is depolarized by an action potential, calcium ions enter the cell during phase 2 of the action potential through calcium channels located on the cell membrane. This triggers a subsequent release of calcium stored in the sarcoplasmic reticulum (SR) through calcium release channels. Calcium released by the SR increases the intracellular calcium concentration with the free calcium binding to the troponin of the regulatory complex attached to the thin filaments. A conformational change results in movement (“ratcheting”) between the myosin and the actin, shortening the sarcomere length. Ratcheting cycles occur as long as the cytosolic calcium remains elevated. At the end of phase 2, calcium entry into the cell slows and calcium is sequestered by the SR via an ATP‐dependent calcium pump, thus lowering the cytosolic calcium concentration and removing calcium from the troponin. To a smaller extent, cytosolic calcium is transported out of the cell by the sodium‐calcium exchange pump. The reduced intracellular calcium induces a conformational change in the troponin complex, leading to inhibition of the actin binding site. Beta‐adrenergic stimulation, which occurs when sympathetic nerves are activated, increases cAMP which in turn activates protein kinase to increase calcium entry into the cell through L‐type calcium channels. ATP, adenosine triphosphate; icAMP, inactive cyclic adenosine monophosphate; PD, phosphodiesterase. The cardiac output (CO) is the driving force for systemic blood flow and is defined as the volume of blood pumped by the heart per unit time. It is a product of the stroke volume × heart rate and can be expressed as liters per minute. Multiple variables can impact each of the components of CO (Figure 11.4) and can contribute to patient compensatory or decompensatory cardiovascular events. Figure 11.4 Factors influencing cardiac output. Cardiac output is the product of heart rate multiplied by stroke volume. Stroke volume (in blue) is the result of the preload, myocardial contractility, and afterload. Vascular tone, venous return, and autonomic innervation are some of the factors that will influence these components. The heart rate (in green) is regulated by autonomic innervation, circulating hormones, and venous return. The overall performance of the heart rests with the stroke volume. This is a delicate interplay between the contractility (inotropic state) of the heart, the afterload (forces opposing the pumping action of the heart), and the preload (forces acting to fill the heart during its quiescent phase). Cardiac performance is also influenced by heart rate, ventriculoatrial coupling, ventricular synchrony, and pericardial properties. The cardiac performance can be further modified by neural control, drugs, hormones, and metabolic products [1]. Preload is the first determinant of contraction (systolic wall motion). It is the force that determines the amount of stretch placed on the contractile elements in the myocardial cell (myocardial sarcomere) at the end of diastole [3]. This increase in diastolic force stretches the sarcomeres and increases the volume in the ventricle at the end of diastole (end‐diastolic volume, EDV). Starling’s law of the heart states that increased sarcomere stretch results in a more forceful contraction, and decreased stretch results in a less forceful contraction [1,4–6]. This gives the heart the ability to regulate stroke volume on a beat‐to‐beat basis and allows the body to acutely increase cardiac output by increasing venous return to the heart [7]. Afterload is the second determinant of wall motion. It opposes muscle shortening and impedes contraction. The forces producing contraction and the forces opposing contraction should be equal and opposite. The pressure that a ventricle generates during systole is usually the same as the systolic systemic arterial blood pressure and is determined by aortic resistance to flow (impedence) [8], stroke volume, and velocity of flow into the aorta. The radius of the systemic arterioles is the primary determinant of resistance and impedance. Afterload is increased when (1) intraventricular pressure is increased (such as arteriolar constriction or aortic stenosis), (2) chamber volume (radius) is increased, or (3) ventricular wall thickness is decreased during systole. The third determinant of stroke volume and systolic wall motion (contraction) is myocardial contractility. Contraction and myocardial contractility are different. Contractility is myocardial performance (force, velocity, and extent of contraction) independent of preload and afterload [4]. Contractility is an inherent myocardial cellular property which, when combined with preload and afterload, influences the force and velocity with which the fundamental contractile unit within the myocardial cell (sarcomere) contracts. Consequently, these factors will change the amount of wall motion or contraction. The primary determinants of contractility include, but are not limited to, (1) increased amount and rate of calcium release by the sarcoplasmic reticulum, (2) increased sensitivity to the released calcium, (3) phosphorylation of proteins within the cell, and (4) cellular adenosine triphosphate (ATP) production [1]. Heart rate (HR) is the number of contractions of the heart per unit time (beats per minute, bpm) and is the remaining component of the CO equation. The normal resting heart rates for the dog and cat are listed in Table 11.1. Tachycardia is a fast heart rate at rest and bradycardia is a slow heart rate at rest. The heart rate can vary according to the physical needs of the patient, to include the need to absorb oxygen and excrete carbon dioxide. Activities that can provoke HR changes include physical exercise, sleep, anxiety, stress, pain, illness, and drugs. An arrhythmia occurs when the heart is not beating in a regular pattern. Table 11.1 Normal resting heart rates for dogs and cats. bpm, beats per minute. Each beat of the heart is set in motion by an electrical signal from within the heart muscle. In a normal, healthy heart, each beat begins with a signal from the sinoatrial (SA) node (pacemaker) located in the right atrium. There are important physiological differences between nodal cells and ventricular cells. The specific differences in ion channels and mechanisms of polarization give rise to the unique spontaneous depolarizations necessary for the pacemaker activity of the SA node. This signal stimulates the atria to contract, and then travels to the atrioventricular (AV) node, located in the interatrial septum. After a delay, the stimulus diverges and is conducted through the left and right bundles of His to the respective Purkinje fibers within each side of the heart, then to the endocardium at the apex of the heart, and finally to the ventricular epicardium (Figure 11.5). The left ventricle contracts an instant before the right ventricle. As the signal passes, the walls of the ventricles relax and await the next signal. This process continues over and over as the atria refill with blood and more electrical signals come from the SA node. Figure 11.5 Schematic of the cardiac electrical conduction system and the corresponding ECG waveforms. The P wave is the result of atrial depolarization which starts in the sinoatrial (SA) node. These signals from the pacemaker cells of the SA node are transmitted to the right and left atria. The impulse is sent to the atrioventricular (AV) node where it is slowed and passed through the His bundle, then through the right and left bundle branches and finally to the Purkinje fibers for myocardial contraction through excitation coupling. The letters Q, R, and S are used to describe the QRS complex and represent ventricular depolarization. The T wave represents repolarization of the ventricles. The criteria for identifying the QRS waves are: Q: the first negative deflection after the P‐wave. If the first deflection is not negative, the Q is absent; R: the first positive deflection; S: the negative deflection after the R‐wave. Intervals are then measured and demonstrated by the colored bars above. The PR interval represents the AV conduction time, measured from where the P‐wave begins to the beginning of the QRS; the QRS interval is measured from the end of the PR interval to the end of the S wave; the QT interval is measured from the beginning of the QRS complex to the end of the T‐wave; indicates time of ventricular activity to include depolarization and repolarization. On the microscopic level, the wave of depolarization propagates to adjacent myocardial cells by way of gap junctions located on the intercalated disks. Electrical impulses propagate freely between cells in every direction. This allows the myocardium to function as a single contractile unit. This property allows rapid, synchronous depolarization of the myocardium. In order to maximize efficiency of contraction and CO, the conduction system of the heart must have the following characteristics: substantial atrial to ventricular delay to allow ventricular filling, coordinated contraction of ventricular cells to provide adequate systolic pressure, and ventricular contraction beginning at the apex of the heart to facilitate upward ejection of blood into the great arteries. After contracting, the heart muscles must relax to fill the chambers again. This relaxation is possible due to rapid repolarization of cardiac muscle caused by a temporary inactivation of certain ion channels. The heart rate is rhythmically generated by the SA node, but is also influenced by central factors mediated through sympathetic and parasympathetic nerves [9]. The medulla oblongata stimulates activity through sympathetic stimulation of the cardioaccelerator nerves, and decreases heart activity through parasympathetic stimulation through the vagus nerve. During rest, both centers provide slight stimulation to the heart, contributing to autonomic tone. Normally, vagal stimulation predominates. The ventricles are more richly innervated by sympathetic fibers than parasympathetic fibers. Sympathetic stimulation causes the release of norepinephrine at the neuromuscular junction of the cardiac nerves. This shortens the repolarization period, speeding the rate of depolarization and contraction. Norepinephrine binds to beta‐1 receptors and opens chemical‐ or ligand‐gated sodium and calcium ion channels, allowing an influx of positively charged ions [10]. The vagus nerve sends branches to both the SA and AV nodes, and to portions of both the atria and ventricles. Parasympathetic stimulation releases acetylcholine at the neuromuscular junction, which slows HR by opening chemical‐ or ligand‐gated potassium ion channels to slow spontaneous depolarization. Repolarization is also extended, increasing the time before the next spontaneous depolarization occurs. The cardiovascular centers receive input from a series of visceral receptors, including proprioreceptors, baroreceptors, and chemoreceptors. Baroreceptors are located in the aortic arch and carotid body and sense tension or stretch in the blood vessels. Decreased intravascular circulating fluid volume is sensed by these receptors, and normal inherent vagal input is decreased. This allows the sympathetic tone to become more prevalent and results in an increased heart rate. The increased heart rate functions to compensate for decreased myocardial contraction from a reduced preload and to maintain cardiac output and blood pressure. With increased pressure and stretch, the rate of baroreceptor firing increases, and the cardiac centers decrease sympathetic stimulation and increase parasympathetic stimulation, slowing the HR. The atrial reflex (or Bainbridge reflex) is stimulated when increased venous return stretches the walls of the atria and stimulates specialized baroreceptors. These atrial baroreceptors increase their rate of firing and stimulate the cardiac center to increase sympathetic stimulation and inhibit parasympathetic stimulation, resulting in an increased HR. The opposite is true when there is a decreased rate of atrial baroreceptor firing. Increased metabolic byproducts associated with increased metabolic activity, such as declining oxygen levels and increased carbon dioxide, hydrogen ions and lactic acid, are detected by chemoreceptors innervated by the glossopharyngeal and vagus nerves. These chemoreceptors provide feedback to the cardiovascular centers about the need for increased or decreased blood flow, based on the relative levels of these substances. Critical illness can place significant metabolic demands on the heart. Problems encountered in the small animal patient, such as hypovolemia, hypotension, hypoxia, and systemic inflammation, can initiate compensatory cardiovascular responses that require an immediate and sustained increase in cardiac output. The heart must be capable of adjusting the heart rate and increasing the myocardical contractile forces to meet the needs for oxygen delivery to the tissues; however, prolonged or excessive tachycardia can result in myocardial hypoxia and ischemia. It is important to identify potential causes of abnormalities in cardiac rate, rhythm or contractility early in the course of treatment and determine the effects that these changes can have on the tissues. Any tissue that requires oxygen can be affected by poor cardiac performance. Unfortunately, no easy procedure is available to directly measure cardiac output in the small animal patient. Instead, historical and physical examination findings, point of care (POC) and clinicopathological laboratory testing, diagnostic imaging and monitoring procedures must be used to gather information that reflects potential causes and effects of cardiac disorders. Patients that have labored breathing or poor perfusion will require therapeutic intervention (such as oxygen supplementation) to support tissue oxygenation throughout the diagnostic period. The history begins with the species and signalment (age, sex, breed). Dogs are more commonly diagnosed with mitral regurgitation and dilated cardiomyopathy as a cause of heart disease, and cats are more likely to have hypertrophic changes causing cardiac disease. Heart disease is diagnosed more commonly in older dogs and cats, with male dogs reported to have an increased incidence of heart disease compared to females. Certain breeds of dogs and cats can have a genetic predisposition to heart disease (Table 11.2). Table 11.2 Breed predilections to cardiac disease. The vaccination and heartworm status should be known and any past diagnosis of a heart murmur, gallop or cardiac arrhythmia reported. Past medical problems can identify disorders such as hyperthyroidism, hypothyroidism, heartworm disease, periodontal disease, malnutrition, diabetes mellitus, cancer or immune‐mediated disorders that can have an effect on cardiac function. A review of prescribed and over‐the‐counter medications and of any exposure to potential toxins might identify a possible cardiotoxic agent. Table 11.3 provides a list of common toxins that can affect cardiac performance in the dog and cat. Table 11.3 Cardioactive toxins. Identifying when the pet was last completely normal helps to determine whether any cardiovascular changes are acute or chronic. A history of trauma, sudden collapse or syncope warrants immediate concern for acute cardiac disorders. Reported clinical signs of coughing, respiratory difficulty, lameness, and exercise intolerance are common presenting complaints with heart, airway, thrombotic or pulmonary disease and suggest a more chronic problem. In the cat, the clinical signs of heart disease can be quite varied and as subtle as anorexia and depression. The physical examination begins with the vital signs (temperature, heart rate, pulse intensity, respiratory rate and effort, mucous membrane color, and capillary refill time (CRT)). Hypothermia can be a consequence of poor perfusion due to insufficient CO and is a common finding in cats. A fever can be an early consequence of valvular endocarditis or systemic inflammatory response syndrome (SIRS) disease with the associated cardiac changes. The presence of sustained tachycardia or bradycardia warrants concern for shock, arrhythmias or poor cardiac performance. The coronary arteries fill during the diastolic phase of the cardiac cycle, and any sustained increase in heart rate could be detrimental. Evaluation of the physical peripheral perfusion parameters (heart rate, pulse quality, mucous membrane color, CRT) can very quickly identify if cardiac output is affecting systemic tissue perfusion. The heart beat and pulse should be synchronous, with irregularities suggestive of an arrhythmia. Distension of the jugular veins can be a result of right heart failure, volume overload, pericardial tamponade or obstruction of the superior vena cava. The breathing pattern is assessed for evidence of lung parenchymal disease, pleural effusion or small airway disease (see Chapter 8). Auscultation of the thorax should begin at the periphery of the thoracic cavity, listening for the softer sounding lung parenchyma and small airways and then moving to more centrally located and louder sounding large airways and heart. Pleural effusion or pulmonary edema can each be a life‐threatening consequence of heart failure. Muffled heart sounds can suggest pericardial fluid or pleural fluid or air accumulation. The heart is auscultated from both sides of the thoracic cavity, with auscultation requiring at least 1–2 minutes of focused listening to rule out the presence of a murmur or gallop heart sound. Closure of the mitral and tricuspid valves (also called AV valves) is associated with the first heart sound and closure of the aortic and pulmonic (semilunar) valves is associated with the second heart sound. When blood inappropriately leaks through an abnormal closed heart valve, it causes turbulence, creating an auscultable murmur. A third heart sound (gallop sound) is often heard in a patient with cardiac disease at or near the peak of rapid ventricular filling. When a murmur is identified, the point of maximum intensity is determined in an effort to isolate the valve most likely diseased (Table 11.4). A murmur can be due to anatomical defects (pathological murmurs) or physiological problems such as anemia, hyperthyroidism, hypertension, fever, and excitement (physiological murmurs). Table 11.4 Abnormal heart sounds with point of maximal intensity and their clinical significance. Ao, aorta; PA, pulmonary artery; LAV, left atrioventricular valve; PMI, point of maximum intensity; RAV, right atrioventricular valve. The timing of the murmur to systole or diastole is recorded. Diastolic murmurs suggest stenosis of the AV valves or regurgitation of the semilunar valves. Causes of systolic murmurs can include stenosis of the semilunar valves, regurgitation of the AV valves, atrial or ventricular septal defects, hypertrophic obstructive cardiomyopathy or physiological flow murmurs. Continuous, combined systolic and diastolic murmurs can be heard with patent ductus arteriosus, coarctation of the aorta, or acute severe aortic regurgitation. The intensity of the murmur is graded, which aids in assessing for changes over time (Table 11.5). Cardiac auscultation must be repeated after intravascular volume resuscitation. Hypovolemia can significantly reduce the volume of regurgitant blood, thereby minimizing or obliterating the sound of the murmur. Restoring the intravascular volume should restore the murmur if valvular regurgitation is present. Table 11.5 How to grade heart murmurs. PMI, point of maximum intensity. Abnormal heart sounds (murmur, gallop) in the cat are frequently intermittent rather than present with each heart beat. This necessitates a focused cardiac auscultation for at least two full minutes to determine whether or not the abnormality is present in the cat. Most feline murmurs have a very focal point of maximum intensity that is generally located just left of the sternum. The neck of the cat should be carefully palpated for evidence of enlarged thyroid glands (thyroid slip), common in hyperthyroid cats (a cause of cardiac disease). The peripheral limbs of the patient are carefully examined for evidence of interstitial edema resulting from cardiogenic changes in the intravascular hydrostatic pressure. The abdomen is carefully palpated to determine if there is hepatomegaly or abdominal effusion, each a potential consequence of right heart failure. A minimum database is provided through POC testing and should include the packed cell volume (PCV), total protein (TP), blood glucose, blood urea nitrogen (BUN) electrolyte panel, blood lactate, blood gas, coagulation profile, blood smear, and urinalysis. As the PCV or hematocrit decreases, blood viscosity decreases and can cause a functional heart murmur. An elevated PCV can be a consequence of chronic hypoxia resulting from cardiac disease (see Chapter 10). Hypoglycemia can affect vascular tone (preload) and myocardial contractility, with insufficient energy substrate available. An elevated BUN and isosthenuria on urinalysis might direct further investigation for kidney disease, a potential consequence of insufficient CO. Electrolyte disorders affecting sodium, potassium, magnesium, calcium or chloride and severe acidemia or alkalemia can each impact cardiac rate, rhythm, myocardial contractility, and vascular tone. Elevated lactate may indicate that there has been poor oxygen delivery to tissues, and resolution of this may be an important parameter to monitor in patients with cardiogenic shock. Evidence of microfilaria may be seen on a blood smear. Heartworm (Dirofilariasis immitis) serology is most commonly performed as a snap test (4DX, Idexx, Westbrook, ME or Accuplex, Antech, Irvine, CA). Current dirofilariasis tests detect antigens only on female heartworms, providing a false‐negative result when there are solely male worms present. A complete blood count (CBC) and serum biochemical profile with thyroid panel will provide data pertaining to possible causes and effect of abnormalities in cardiac rate, rhythm or myocardial contractility. The CBC can better define anemia or erythrocytosis when present. An elevation in serum creatinine and BUN directs further investigation for kidney disease and possible renal hypertension which can affect preload and afterload. Elevated liver enzymes can occur with heartworm infestation or hepatic congestion from right heart disease. Hyperthyroidism and hypothyroidism can each affect cardiac performance. Feline patients with chronic thyrotoxicosis may have “masked” renal injury (due to increased renal blood flow), requiring close monitoring of renal parameters during treatment. Additional testing is often required and could include serology for inciting pathogens (cause of SIRS‐related cardiac changes) and fluid analysis with culture and susceptibility. Many of the drugs that are administered for cardiac disease (such as digoxin, furosemide, angiotensin converting enzyme (ACE) inhibitors) require baseline bloodwork and urinalysis at the start of therapy to help avoid side‐effects and to tailor drug therapy for the individual patient over time. Several cardiac biomarkers (such as cardiac troponin I, NT‐pro‐BNP) are used in human medicine to determine if cardiac injury is immediately present. The significance of these biomarkers in the diagnosis and treatment of heart disease in the dog and cat has not been completely elucidated. Their usefulness might be in differentiating cardiac from noncardiac disease or determining if significant cardiac disease is present prior to the onset of heart failure [11–17]. In addition, these biomarkers might be used to monitor changes in the heart over time [16,18]. Cardiac troponin I and NT‐pro‐BNP have also been investigated as prognostic indicators in dogs with dilated cardiomyopathy [19] and dogs with myxomatous mitral valve disease [20]. However, these test results should not be used as a sole diagnostic or prognostic indicator. While elevation in the test results might indicate myocardial injury, the results do not differentiate the etiologies of myocardial injury [15,21,22]. However, two studies have demonstrated that elevated NT‐pro‐BNP can be supportive of a cardiac cause of respiratory distress in cats [23,24]. Imaging of the cardiovascular system is an essential component of cardiac evaluation. Imaging most commonly involves thoracic radiographs and echocardiography, including color flow Doppler, and electrocardiography (ECG). Occasionally ultrasound imaging will be used to assess the size and patency of vessels for determining blood flow. Survey thoracic radiographs are reviewed for evidence of cardiac disease. Radiographic findings that are suggestive of cardiac disease are listed in Table 11.6. The thoracic radiographs can be used to determine the vertebral heart score, performed to document the presence or absence of cardiomegaly. The procedure for assessing this heart score is outlined in Box 11.1. Table 11.6 Survey radiograph findings suggestive of cardiac disease in the dog and cat. CHF, congestive heart failure; LA, left atrium; VHS, vertebral heart score; Ultrasound imaging of the heart and great vessels (termed echocardiography) has changed the way in which cardiac anatomy is clinically evaluated and is complementary to radiographic imaging. The rapid rate of image acquisition allows evaluation of cardiac motion over time, making it possible to evaluate cardiac function noninvasively. Doppler echocardiography (spectral and color flow Doppler) adds the ability to evaluate blood flow direction and velocity and patterns of blood flow within the heart and great vessels. Transesophageal echocardiography is increasingly used in human intensive care medicine but has not yet become widely available in veterinary medicine. Because echocardiography can demonstrate cardiac anatomy, cardiac function, and blood flow patterns, it has supplanted cardiac catheterization as the preferred method for critically evaluating the heart in clinical veterinary medicine. Although echocardiography is a powerful diagnostic aid, it should not be used in isolation. Rather, it must be considered as one component of a complete cardiovascular examination [1]. The two‐dimensional examination usually begins on the right side of the thorax and proceeds to the left caudal and ultimately left cranial views. Long axis and short axis view planes can be consistently obtained in most dogs and cats (Figures 11.6, 11.7, and 11.8). The examination can demonstrate the chamber sizes of the heart, wall thickness and movement, valvular movement and lesions, fractional shortening, pericardial changes, and pleural space abnormalities. Normal values for the dog are dependent on the body weight, with normograms provided in the software by the manufacturer of most veterinary ultrasound equipment. Normal values for the cat are listed in Box 11.2. Detailed information regarding how to perform a two‐dimensional and M‐mode echocardiogram and Doppler flow studies can be found in the references. Figure 11.6 Long axis echocardiography views. With the beam plane oriented nearly perpendicular to the long axis of the body, parallel to the long axis of the heart, and with the transducer index mark pointing toward the heart base (dorsal), two views are usually obtained. The first is a four‐chamber view with the cardiac apex (ventricles) displayed to the left and the base (atria) to the right. The second, obtained by slight clockwise rotation (when viewed from the bottom of the transducer) of the transducer from the four‐chamber view into a slightly more craniodorsal‐to‐caudoventral orientation, shows the left ventricular outflow tract, aortic valve, aortic root, and proximal ascending aorta. Source: Kittleson MD, Kienle RD, eds. Normal clinical cardiovascular physiology. In: Small Animal Cardiovascular Medicine. St Louis: Mosby. 1998. Used with permission of Elsevier [1]. Figure 11.7 Schematic representations of the long axis echocardiography views obtained from the right parasternal window. The black circle at the top of the sector indicates the index mark. Source: Keinle RD, Thomas WP. Echocardiography. In: Veterinary Diagnostic Ultrasound. Nyland T, Mattoon J, eds. Philadelphia: WB Saunders, 1995. Used with permission of Elsevier. AO, aorta; LA, left atrium; LC, left coronary cusp of the aortic valve; LV, left ventricle; RPA, right pulmonary artery; RV, right ventricle. Figure 11.8 Short axis echocardiography views. By rotating the transducer approximately 90° clockwise from the four‐chamber view so that the beam plane is oriented close to the long axis of the body and perpendicular to the long axis of the heart and the transducer index mark is pointing cranially (or cranioventrally), a series of short axis views are obtained. Proper short axis orientation is identified by the circular symmetry of the left ventricle or aortic root. Short axis planes are commonly obtained at the level of the left ventricular apex, papillary muscles, chordae tendineae, mitral valve, and aortic valve by angling the beam from the apex (ventral) to the base (dorsal). In most animals, further dorsal angulation and slight rotation allow imaging of the proximal ascending aorta, right atrium, and pulmonary artery branches. The images should be displayed with the cranial part of the image to the right and the right heart encircling the left ventricle and aorta clockwise (the right ventricular outflow tract and pulmonary valve to the right). Source: Kittleson MD, Kienle RD, eds. Normal clinical cardiovascular physiology. In: Small Animal Cardiovascular Medicine. St Louis: Mosby. 1998. Used with permission of Elsevier [1]. AMV, anterior mitral valve leaflet; AO, aorta; APM, anterior papillary muscle; AV, aortic valve; CaVC, caudal vena cava; CH, chorda tendineae; LA, left atrium; LC, left coronary cusp of the aortic valve; LPA, left pulmonary artery; LV, left ventricle; LVO, left ventricular outflow tract; MV, mitral valve; NC, noncoronary cusp of the aortic valve; PA, pulmonary artery; PM, papillary muscle; PMV, posterior mitral valve leaflet; PPM, posterior papillary muscle; PV, pulmonary valve; RA, right atrium; RC, right coronary cusp of the aortic valve; RPA, right pulmonary artery; RV, right ventricle; RVO, right ventricular outflow tract; TV, tricuspid valve; VS, interventricular septum. Preload, afterload, and contractility determine systolic wall motion and are assessed by the amount of wall contraction seen on an echocardiogram. This is measured by calculating the fractional shortening (FS%). When examining how these factors change in cardiovascular disease, the change in the EDV of the ventricle, the end‐systolic volume (ESV) of the ventricle, the wall thickness, and the ability of the ventricle to eject blood into the aorta are determined [1]. The formula for calculating the FS% is presented in Box 11.3, with the result used as an indication of ventricular performance. These variables have been illustrated on the M‐mode echocardiogram tracing shown in Figures 11.9 and 11.10. Normal canine long axis echocardiographic images can be found in Figure 11.11 and short axis images in Figure 11.12. Two‐dimensional echocardiographic images in the normal cat are illustrated in Figure 11.13. Figure 11.9 Variables of M‐mode echocardiography. Recommended methods for echocardiographic measurements from the M‐mode echocardiogram. The standard M‐mode sweep from the level of the left ventricular papillary muscles to the level of the aorta and left atrium, showing the locations and timing of chamber dimension and wall thickness measurements. Source: Keinle RD, Thomas WP. Echocardiography. In: Veterinary Diagnostic Ultrasound. Nyland T, Mattoon J, eds. Philadelphia: WB Saunders, 1995. Used with permission of Elsevier. For abbreviations see Figures 11.6 and 11.7. Figure 11.10 M‐mode echocardiographic images from a normal dog and cat. (a) Normal canine M‐mode echocardiograms recorded at the level of the (1) left ventricle, (2) mitral valve, and (3) aorta and left atrium. (b) Normal feline M‐mode echocardiogram recorded at the same levels as in (a) (arrow indicates the aortic valve). Source: Keinle RD, Thomas WP. Echocardiography. In: Veterinary Diagnostic Ultrasound. Nyland T, Mattoon J, eds. Philadelphia: WB Saunders, 1995. Used with permission of Elsevier. For abbreviations see Figures 11.6 and 11.7. Figure 11.11 Long axis echocardiographic images from a normal dog. (a) Right parasternal four‐chamber view. The arrow identifies the fossa ovalis, which may falsely appear to be a septal defect. (b) Right parasternal long axis view. (c) Left caudal (apical) parasternal four‐chamber view. (d) Left caudal (apical) parasternal long axis view (five‐chamber view) [25]. For abbreviations see Figures 11.6 and 11.7. Figure 11.12 Short axis echocardiographic images from a normal dog. (a) Right parasternal short axis view at the level of the left ventricular papillary muscles. (b) Right parasternal short axis view at the level of the mitral valve. (c) Right parasternal short axis view at the level of the aortic valve. (d) Left cranial parasternal short axis view at the level of the aortic valve [25]. For abbreviations see Figures 11.6 and 11.7. Figure 11.13 Two‐dimensional echocardiographic images from a normal cat. (a) Right parasternal four‐chamber view. (b) Right parasternal short axis view at the level of the left ventricular papillary muscles. (c) Right parasternal short axis view at the level of the aortic valve. (d) Left caudal (apical) parasternal four‐chamber view [25]. For abbreviations see Figures 11.6 and 11.7.
Heart rate, rhythm, and contractility
Introduction and physiology of heart rate, rhythm, and contractility
Species
Normal
(bpm) resting
Tachycardia
(bpm) resting
Bradycardia
(bpm) resting
Dog – small breeds
60–160
>180
<90
Dog – medium size
60–160
>160
<60
Dog – large breed
60–160
>160
<60
Cat
150–220
>220
<120
Initial diagnostic and monitoring procedures
History
Disease
Breed/signalment/disease predilection
Dilated cardiomyopathy
Doberman Pinscher, Boxer, Great Dane, Labrador Retreiver, American Cocker Spaniel, large hounds, St Bernard, Old English Sheepdog, cats fed a taurine‐deficient diet
Myxomatous mitral valve disease
Cavalier King Charles Spaniel; small breeds
Hypertrophic cardiomyopathy
Uncommon in dogs, common in cats; seen in cats with hyperthyroidism
Restrictive and unclassified cardiomyopathy
Cats
Zootoxins
Bufo toads (cardioactive glycoside)
Puffer fish (tetrodotoxin)
Anemones and jelly fish (Cnidarians, Na and K channel blockers)
Snake envenomation (uncommon)
Plant toxins
Foxglove
Milkweed
Kalanchoe
Lilly of the valley
Oleander
Star of Bethlehem
Yew
Nicotine
Methylxanthines
Chocolate
Terbutaline/Aminophylline
Human neurological medications
Antipsychotics
Antidepressants
ADHD medications
Common cardiac, asthma or antiarrhythmic medications
See Table 11.13
Beta‐agonists (albuterol)
Illicit/other drugs
Cocaine
Amphetamines
Alcohol
Physical examination
Abnormal heart sound
Significance
Murmur, PMI over the PA valve
May indicate pulmonic stenosis
Murmur, PMI over the Ao valve
May indicate aortic stenosis
Murmur, PMI over the RAV valve
May indicate tricuspid valve insufficiency
Murmur, PMI over the LAV valve
May indicate mitral valve insufficiency
Gallop
May indicate cardiomyopathy
Muffled sounds
May indicate pleural or pericardial fluid or mass
Sounds not synchronous with pulses
May indicate arrhythmia
Grade
Characteristics
I
Minimally auscultable with a stethoscope
II
Soft, but easily heard with a stethoscope
III
Intermediate or moderate loudness with a stethoscope
IV
Loud murmur with sound that radiates to other areas of the chest (PMI may be difficult to determine), no thrill (vibration) palpated
V
Very loud with stethoscope barely touching chest, a palpable thrill (vibration) is present
VI
Very loud with stethoscope, is audible with or without stethoscope, palpable thrill (vibration of chest wall) is present
Point of care testing
Clinicopathological laboratory testing
Diagnostic imaging
Disease
Radiographic findings
Dilated cardiomyopathy
Enlarged heart (VHS >10.7 in a dog), + evidence of CHF, enlarged LA
Hypertrophic cardiomyopathy
May be normal, when severe, cardiomegaly and LA enlargement; valentine‐shaped heart and evidence of CHF
Mitral valve disease
Enlarged heart (VHS >10.7 in a dog), + evidence of CHF, enlarged LA
HWD (Dirofilaria immitis)
Right ventricular and atrial enlargement, dilated and tortuous pulmonary arteries, interstitial changes to lungs
Pericardial effusion
Globoid appearance to heart
Pulmonary hypertension
May be normal; right heart enlargement
Pulmonary thromboembolism
May be normal, right side of heart may be enlarged, attenuation (small size) of pulmonary arterial vessels
Congestive heart failure, CHF (dog)
Left atrial enlargement, cardiomegaly, perihilar interstitital to alveolar pulmonary pattern
Congestive heart failure, CHF (cat)
Cardiomegaly may or may not be present, pleural effusion may or may not be present, pulmonary edema may present as variable, diffuse or patchy interstitial to alveolar pattern
Echocardiography
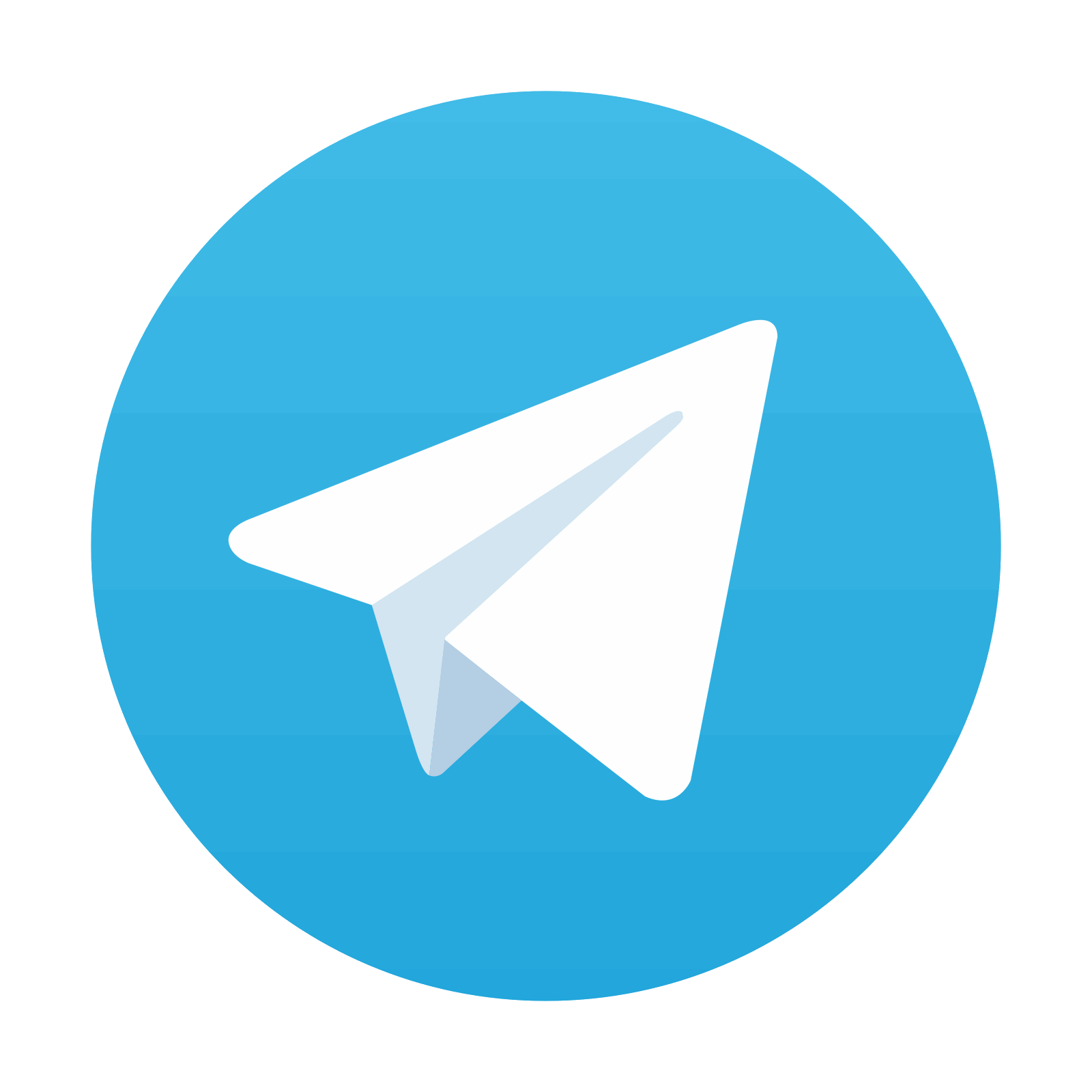
Stay updated, free articles. Join our Telegram channel

Full access? Get Clinical Tree
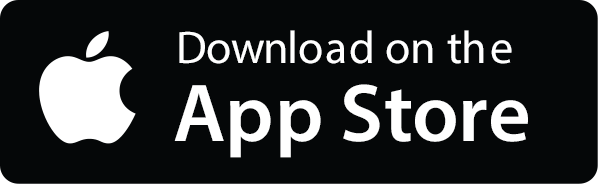
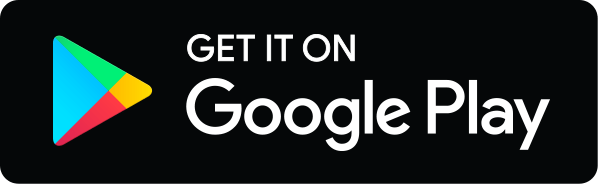