CHAPTER 10 Andrew Linklater1 and Veronica Higgs2 1 Lakeshore Veterinary Specialists, Milwaukee, Wisconsin 2 Metropolitan Veterinary Specialists and Emergency Services, Louisville, Kentucky Alterations in the red blood cell (RBC or erythrocyte) and hemoglobin (Hgb) content of the blood are common in the critically ill small animal patient. Changes in these blood parameters can occur as part of the primary disease or, potentially, as a complication of diagnostic testing or treatment. The effects can cause life‐threatening problems due to impaired perfusion and poor tissue oxygenation, warranting diligent monitoring with timely intervention for a successful patient outcome. Erythrocytes or RBCs consist mainly of Hgb, a complex metalloprotein. The iron (Fe) atoms of the four heme groups (tetramers) of the Hgb molecule temporarily bind to oxygen molecules. Inhaled oxygen easily diffuses from the alveoli through the cell membrane of the erythrocyte as the cell traverses the pulmonary capillaries. The majority of oxygen found in arterial blood is bound to Hgb, with a much lesser amount dissolved in the plasma (Box 10.1). This makes the RBCs and Hgb molecules critical to the delivery of oxygen (DO2) to the tissues. In addition to oxygen transport, the Hgb molecule will transport a portion of the carbon dioxide from the tissues to the lungs for exhalation. The RBC is made in the bone marrow by a process called erythropoiesis, and is under the control of the hormone erythropoietin (EPO). Juxtaglomerular cells in the kidney produce EPO in response to decreased oxygen delivery (as in anemia and hypoxia) and increased levels of androgens. The mechanism of oxygen concentration detection is through hypoxia inducible factor 1 which is a transcription activator that is oxygen sensitive. The RBC development process is controlled and influenced by a number of different factors, including EPO, cytokines (interleukin (IL)‐3 and IL‐4), and granulocyte‐macrophage colony stimulating factor. In addition to EPO, RBC production requires adequate supplies of substrates, mainly iron, vitamin B12, and folate. During the initial phase of erythrocyte production, the multipotential myeloid stem cell differentiates into the erythroid stem cell. From here, the first erythrocyte precursor, the proerythroblast (also called rubriblast), is formed, which has a nucleus and is under the influence of EPO. This cell becomes the basophilic erythroblast, which gains its color from the ongoing production of basophilic staining Hgb in the cytoplasm. This cell transforms into the last RBC precursor capable of mitosis, the smaller polychromatic erythroblast (or prorubricyte). The next stage, called the normoblast (or rubricyte), finds the cell smaller with a small dense nucleus and not capable of mitosis. The reticulocyte (metarubricyte) follows, which has extruded the nucleus and has a slight basophilic staining and webbing of the cytoplasm. These cells can carry oxygen and can normally be found in small numbers in the bloodstream. The final product of erythropoiesis is the erythrocyte or RBC, which is released from the bone marrow into the circulating bloodstream. The shape of the mature RBC is a biconcave disk, depressed and flattened in the center. This distinctive shape optimizes the flow properties of blood in the large vessels. The membrane of the RBC plays many roles that aid in regulating their surface deformability, flexibility, adhesion to other cells, and immune recognition. These functions are highly dependent on its three‐layer composition: the carbohydrate‐rich glycocalyx on the exterior; the lipid bilayer containing transmembrane proteins; and the membrane skeleton, a structural network of proteins located on the inner surface of the lipid bilayer. The mature RBC diameter is approximately 7 microns in the dog, with a life span of 110–120 days. In the cat, the RBC diameter is 5.8 microns with a life span of 65–76 days. The normal RBC count in the dog is 4.8–9.3 million/microliter with a packed cell volume (PCV) of 35–55%. In the cat, the normal RBC count is 5.91–9.93 million/microliter with a PCV of 29–45%. Healthy dogs will have <1.5% reticulocytes and healthy cats <1% reticulocytes. Old RBCs are removed from circulation by the spleen and liver. The spleen acts as a filter for the blood, recognizing and removing old, malformed, or damaged RBCs. The RBCs must pass through a maze of narrow passages, with healthy blood cells simply passing through and continuing back into the circulation. Most old or damaged RBCs are removed from the bloodstream by phagocytosis. The iron is stored as either ferritin or bilirubin, and eventually returns to the bone marrow where Hgb is made. The spleen will also act as a storage unit for blood. The canine and feline spleen both have a large storage capacity, estimated as 10–20% of the circulating RBC mass. Within the spleen there are three pools described in which the RBCs circulate. The majority (~90%) of the circulating blood enters the rapid pool and traverses through the organ in about 30 seconds. Approximately 9% of the red blood cells enters the intermediate pool and circulates through within eight minutes. The remaining 1% traverses through the slow pool, which can take nearly an hour to complete its circulation through the spleen. Under conditions of stress, hypoxemia, heavy exercise, and excessive blood loss, up to 98% of the splenic blood volume is shifted to the rapid pool through splenic contraction to significantly increase the circulating blood volume. The interaction of splenic innervation and adrenal medullary hormones appears to be responsible for splenic contraction in the cat and dog [1,2]. Disorders of Hgb or RBC content in the circulating blood can be categorized as anemia, when there is a low RBC count and Hgb content, erythrocytosis, when there is an elevated RBC count and Hgb content, or dyshemoglobinemia, when there are abnormalities in the hemoglobin molecule. Many of the abnormalities associated with RBCs and Hgb content can be identified through the history, physical examination, and other diagnostic testing tools. Monitoring the critical small animal ICU patient for signs associated with altered RBC and Hgb content is essential. The majority of diagnostic data used for detecting the presence of anemia or erythroctosis can be obtained through the history, physical examination, and cage‐side point of care (POC) testing. The rapid return of POC diagnostic information can be used to select and guide any emergent therapy that may be required. Additional clinicopathological laboratory testing and imaging may be necessary to make a definitive diagnosis of the underlying disease process. The history begins with the signalment (breed, age, sex). Any possible breed‐related problems are considered, such as sighthounds with higher PCVs,; pyruvate kinase deficiency in Basenjis, Beagles, and Cairn Terriers; stomatocytosis in Alaskan Malamutes; hemolytic anemia in American Cocker Spaniels; and phosphofructose kinase deficiency in English Springer Spaniels. Past medical history should highlight vaccination status, indoor or outdoor activity (exposure to infectious disease), retroviral status in cats, and possible exposure to toxins. A list of common drugs and toxins that can cause anemia in the dog and cat is provided in Table 10.1. Chronic administration of diuretics could cause dehydration and erythrocytosis. Drugs such as nonsteroidal antiinflammatory drugs (NSAIDs) or glucocorticosteroids could cause gastrointestinal (GI) ulceration and anemia from blood loss. The excessive supplementation of zinc or the administration of acetaminophen could be a cause for anemia. Recent halothane anesthesia can cause sequestration of RBCs in the spleen and other body areas in the dog [3]. Table 10.1 Common drugs and toxins associated with anemia in the dog and cat. The category of the mechanism of anemia is in bold. NSAIDs = nonsteroidal anti‐inflammatory drugs, DIC = disseminated intravascular coagulation, EPO = erythropoietin. DIC, disseminated intravascular coagulation; EPO, erythropoietin; NSAID, nonsteroidal antiinflammatory drug. The travel history can warrant suspicion of exposure to infectious agents (such as babesiosis) that can cause anemia. A recent history of intestinal parasites might indicate a hookworm or whipworm infestation as a cause of blood loss anemia. The altitude where the patient lives is important since the lower oxygen of higher altitudes results in a higher PCV in normal dogs and cats. Semimoist cat food contains propylene glycol that can cause oxidant injury and Heinz bodies in the RBC of the cat. Although these cats are not usually anemic, RBC life span is reduced. The consequences of anemia or erythrocytosis are often quite apparent to owners, with lethargy, inappetance, increased respiratory rate, weakness, collapse, yellow discoloration to skin or blood in the urine or feces as features in the presenting complaint. Behavioral changes, neurological abnormalities noted at home or changes in energy level can be a complaint associated with either anemia or erythrocytosis. The observation of frank red blood in the vomitus or stool, black, tarry stool or “coffee ground” flecks in the vomitus suggests GI bleeding as a source of RBC and Hgb loss. Abnormal urine color could be indicative of hemoglobinuria or bilirubinuria secondary to RBC lysis. Physical examination begins with the temperature, pulse rate and intensity, respiratory rate and effort, mucous membrane color, and capillary refill time (CRT). Table 10.2 lists common abnormal physical examination findings associated with anemia and erythrocytosis. Table 10.2 Common physical examination findings occurring with abnormal erythrocyte and hemoglobin concentrations. Basic physical examination parameters can vary dramatically depending on the rate of RBC or Hgb changes. Physical signs of anemia and dyshemoglobinemia are associated with poor oxygen delivery to the tissues and can include tachycardia, increased respiratory rate, altered mentation, severe lethargy, weakness, weak pulses, pale or white mucous membranes, and prolonged or absent CRT. Patients with dyshemoglobinemias may have muddy or brown skin or mucous membranes when there is methemoglobinemia or brick red mucous membranes with carboxyhemoglobinemia. The blue tongue and membrane coloration of cyanosis is evident when there is 5 g/dL of deoxygenated Hgb. The brown coloration of methemoglobin requires only 1.5 g/dL of deoxygenated Hgb. Cardiac murmurs may be noted on auscultation of an animal with anemia or erythrocytosis due to the changes in blood rheology and turbulence of the cardiac blood flow. Icterus noted in the skin and mucous membranes, along with pigmenturia, may be seen in patients with RBC hemolysis. The oral cavity, mucous membranes, integument, and rectal examination can provide physical evidence of bleeding. While blood loss through petechiae, ecchymoses or bruising is impossible to quantify, substantial quantities of RBCs can be lost within these affected tissues. Areas of bruising or ecchymoses in the skin should be outlined with a waterproof nontoxic marker to monitor for changes in size over time. Any skin or subcutaneous abnormal swellings or masses could be related to hemorrhage and should be outlined with a marker and monitored for progression. A thorough exam for external parasites can reveal a heavy infestation of fleas or ticks that can cause a severe anemia. The abdomen is palpated and balotted for fluid waves. A positive ballotment of the abdomen suggests that there is a large volume of fluid within the cavity. The abdomen and limbs can be measured and the circumference tracked for evidence of expansion that could be due to internal bleeding. The site of collection of blood samples must be considered and commonly includes small veins or capillary beds (such as clipped nails or ear veins) for microhematocrit tubes and peripheral (such as cephalic or saphenous veins) or central veins (such as the jugular vein or through a central catheter) for larger volumes of blood. Using a central catheter for blood collection can avoid repeated entry into a vein by a needle. The three‐syringe technique (Box 10.2) is used for blood collection from a catheter to minimize blood loss and to avoid iatrogenic dilution of blood samples due to the catheter flushing solution. The POC testing should include the packed cell volume (PCV), total protein (TP), blood glucose, blood urea nitrogen (BUN), electrolytes, acid–base status, coagulation profile, blood smear, blood lactate, fecal exam for parasites, and urinalysis. Normal values for PCV/TP, Hgb, and other complete blood count (CBC) values are noted in Table 10.3 [4]. Table 10.3 Normal laboratory values for the dog and cat [4].* * Values will vary between laboratory analyzers. When indicated, saline agglutination testing is done to identify autoagglutination as a cause of RBC intravascular hemolysis. Cross‐match testing kits (Rapidvet®‐H, DMS Laboratories Inc., Flemington, NJ) are available for POC testing prior to the administration of a blood transfusion. Canine and feline blood typing (Rapidvet®‐H, Dms Laboratories Inc., Flemington, NJ) agglutination test cards are also available as POC testing. The PCV and spun hematocrit (Hct) are interchangeable names for the simple and inexpensive method of assessing the percentage of centrifuged anticoagulated whole blood in a microhematocrit tube, that is, RBCs. The PCV result can normally vary by up to 5% between measurements. The true hematocrit is technically a value calculated by automated hematology analyzers, using the formula: MCV × RBC count/10, where MCV is the mean corpuscular volume. Spectrophotometry has also been used to determine the hematocrit value. The PCV and Hct will each provide data for identifying anemia or erythrocytosis. Trends of change should be monitored by consistently using the same method (PCV or calculated Hct) throughout hospitalization. The TP and state of hydration of the patient are important when evaluating the significance of changes in the PCV or Hct. Common alterations observed in the PCV and TP with anemia and erythrocytosis and possible etiologies are listed in Table 10.4. Transient erythrocytosis can occur when a dog is anxious or excitable due to splenic contraction. This transient increase in RBCs is particularly noticeable in large breed dogs and usually subsides within one hour [5]. Splenic contraction may occur in response to hemorrhage to maintain a normal PCV. However, there is no reserve for protein, causing a relative decrease in TP compared to the PCV. Both the PCV and TP will continue to drop after hemorrhage due to fluid resuscitation and redistribution of body fluids. Table 10.4 Possible mechanisms of anemia and polycythemia related to changed in the PCV and TP. N, normal; PCV, packed cell volume; TP, total protein; w/= with. It is also important to observe the size of the buffy coat (white blood cells and platelets) and serum color (straw colored = normal, red = hemoglobin or less likely, myoglobin, white = lipemia, yellow = icterus) of the spun microhematocrit tube. Icteric or hemolyzed serum may indicate RBC destruction. An elevation in BUN can be a reflection of renal disease, which can impact EPO production and the occurrence of anemia. BUN may also be elevated with dehydration (prerenal azotemia), so PCV or Hct may be falsely elevated. In addition, the presence of free Hgb can cause renal failure. However, blood within the GI tract can also cause an increase in BUN. A low BUN warrants further investigation for liver dysfunction, which is a potential cause of anemia. Hyperglycemia can cause severe dehydration that can increase the PCV and TP results. Hyperkalemia can be seen with the destruction of RBCs in the Akita. Hypophosphatemia can cause lysis of the RBC cell membrane. The presence of thrombocytopenia or a coagulopathy can cause blood loss anemia. Erythrocytosis can be associated with a hypercoagulable state (see Chapter 9). The carbonic anhydrase within the RBC plays a vital role in the rapid response to respiratory acidosis. The acid–base balance appears to be corrected by reduction of the RBC carbonic anhydrase activity in the case of alkalosis, and by an increase in RBC carbonic anhydrase activity in the case of acidosis [6]. Elevations in blood lactate provide an assessment of anaerobic metabolism in the tissues. Elevated lactate levels at presentation (4.8 mmol/L vs 2.9 mmol/L) have been associated with nonsurvival in dogs with immune‐mediated hemolytic anemia [7]. However, a change in lactate over time is of more predictive value than a single admission lactate. Evaluation of the urine specific gravity provides insight into the concentrating abilities of the kidney, with isosthenuria suggestive of renal disease. Hematuria can be microscopic or gross and a source of chronic blood loss. However, hematuria rarely causes blood loss significant enough to be the primary cause of anemia. Hemoglobinuria (free Hgb) causes red coloration of the urine identical to hematuria. However, when the urine is centrifuged, the supernatant will be clear with hematuria and remain red with hemoglobinuria. Bilirubinuria provides a greenish brown coloration to the urine and can be a consequence of extravascular hemolysis or other causes of icterus. The microscopic examination of a fecal preparation is performed to detect hookworms or whipworms that can be an important source of blood loss. A blood smear allows an initial assessment of the RBC morphology. A search is made for the bone marrow response and any underlying cause of anemia. A list of the common microscopic changes in the erythrocyte and brief interpretation of the finding is provided in Table 10.5. Blood collected for detecting Mycoplasma in the cat is best sampled from a peripheral vein and smeared immediately on a glass slide to maximize the potential identification of these organisms. When searching for Babesia, blood is collected from a peripheral capillary, such as the ear pinna, and smeared and stained immediately. A search of the RBC membrane surfaces and interior is made for the presence of bacteria or parasites that might be the cause of the RBC disorder. Table 10.5 Microscopic erythrocyte changes and associated etiologies for anemia. DIC, disseminated intravascular coagulation; FeLV, feline leukemia virus; RBC, red blood cell. The color of erythrocytes is due to the heme group of hemoglobin. When combined with oxygen, the resulting oxyhemoglobin is scarlet, and when oxygen has been released the resulting deoxyhemoglobin is of a dark red burgundy color. Low numbers (<10%) of single, small Heinz bodies may be seen on erythrocytes in healthy cats. These “endogenous” Heinz bodies may be seen in increased numbers (up to 50%) in cats on semimoist food that contains propylene glycol that causes oxidant injury. Healthy cats also can have a few Howell–Jolly bodies in erythrocytes in peripheral blood. In large blood vessels, RBCs sometimes occur as a stack, flat side next to flat side. This is known as rouleaux formation, and it occurs more often if the levels of certain serum proteins are elevated, such as during inflammation. A saline agglutination test is an easy and inexpensive way to screen for autoagglutination and should be a part of the POC evaluation of anemia. The steps for performing a saline agglutination test are outlined in Box 10.3. Agglutination of the RBCs must be differentiated from rouleaux formation. This evaluation can also be used to assess response to therapy when the saline agglutination is positive. A CBC, with review by pathologist, and serum biochemical profile are submitted to characterize changes in the RBCs, identify an underlying cause, and assess for consequences of disorders of RBCs and Hgb. Additional testing can include bone marrow aspirate and core biopsy, serum titers for infectious diseases, and Coombs testing for immune‐mediated anemia. The white blood cell (WBC), RBC, and platelet counts are assessed to determine whether the disorder affects other cell lines. The total WBC count must be corrected for the presence of circulating nucleated RBCs (rubricytes) when they are present. Hemoglobin is measured by a spectrophotometer in a suspension of lyzed RBCs. Substances, such as lipemia, that increase the optical density of the solution can cause spuriously high readings. The numerical value for Hgb should be approximately one‐third of the PCV value [8]. The MCV and mean corpuscular Hgb concentration (MCHC) are measured with the CBC and normal values are provided in Table 10.3. The MCV is a measure of the average volume of a RBC. In patients with anemia, it is the MCV measurement that allows classification as a microcytic anemia (low MCV; chronic disease, blood loss, iron deficiency), normocytic anemia (MCV within normal range) or macrocytic anemia (high MCV; B12 and folic acid deficiencies). Normocytic anemia is typically seen in acute conditions (blood loss, hemolysis) when the bone marrow has not yet responded with a change in cell volume. The MCHC is a measure of the concentration of Hgb in a given volume of packed RBCs. MCHC is diminished or hypochromic in microcytic anemias, and normochromic in macrocytic anemias. When anemia is present, the RBC morphology is examined for evidence of a bone marrow response (regeneration). It may take 3–5 days for a normal regenerative response from the bone marrow to be recognized. The presence of polychromasia, reticulocytosis, anisocytosis, macrocytosis, and hypochromasia can support regeneration. The reticulocyte count can be used to determine the degree of regeneration (Box 10.4). Special staining with new methylene blue is required to identify the reticulocytes (Figure 10.1). The absolute reticulocyte count is a percentage of RBCs that are reticulocytes. However, as Hgb drops and anemia is persistent, several things happen: (1) reticulocytes are mixed with a lower percentage of circulating mature erythrocytes, (2) immature reticulocytes are released earlier (called shift reticulocytes), and (3) reticulocytes persist longer in circulation; these result in a falsely high impression of reticulocytes with an absolute count. Corrected reticulocyte percentage and absolute reticulocyte counts correct for (1); reticulocyte production index (in dogs) corrects for (1), (2), and (3) [9]. The significant reticulocyte values and formulas for these corrections are provided in Box 10.4. While nucleated red blood cells may be noted with regeneration, their presence is not a reliable indicator of a regenerative response. The cat has two kinds of reticulocytes: aggregate reticulocytes and punctate reticulocytes. Punctate reticulocytes can remain in circulation for up to three weeks. It is the aggregate reticulocytes that must be counted when evaluating anemia for a current regenerative response. Aggregate reticulocytes can be identified by their linear aggregates of ribosomes, rather than individual (punctate) structures as seen in punctate reticulocytes (see Figure 10.1). Figure 10.1 An image of canine (top) and feline (bottom) reticulocytes stained with new methylene blue. Feline aggregate (black arrow) and punctate (white arrow) reticulocytes are shown. Source: Courtesy of Dr Karen Young, University of Wisconsin‐Madison. The serum biochemical profile is evaluated for evidence of hepatic or renal disorders, hypoalbuminemia (or hypoproteinemia), hypophosphatemia (as a cause of hemolysis), and hyperbilirubinemia. The finding of renal disease can implicate impaired production of EPO as a source of RBC problems, or implicated free Hgb, when present, as a cause. Chronic disease of any etiology can have anemia as a consequence. Hypothyroid patients can also develop an anemia. PCR or serum testing for RBC parasites may also be useful in select patients or geographic regions. Further coagulation testing may be indicated to identify an occult source of hemorrhage. A positive D‐dimer or fibrin split product test and low or falling plasma fibrinogen concentration are highly suggestive of disseminated intravascular coagulation [11]. Individual factor testing for congenital or acquired hemophilia and von Willebrand’s disease screening may be indicated as well (see Chapter 9). Serum levels of erythropoietin may be indicated if an absolute erythrocytosis or an unexplained nonregenerative anemia is found. Toxicology screening can diagnose heavy metal, rodenticide, acetaminophen or other toxicities causing anemia. Co‐oximetry may be necessary to further evaluate dyshemoglobinemias. Iron is a necessary metal required not only for the synthesis of hemoglobin but also for many cellular enzymes and co‐enzymes. Iron is transported in serum bound to the protein transferrin. Normally, only about one‐third of the available binding sites on transferrin are occupied by iron. The total iron binding capacity is found in serum; therefore, it includes the amount of iron already bound to the transferrin (serum iron) plus the amount of iron required to saturate the unoccupied binding sites of transferrin. However, because the liver produces transferrin, alterations in function (such as cirrhosis, hepatitis, or liver failure) must be considered when performing this test. The determination of serum iron total iron binding capacity (TIBC) and percent transferrin saturation is useful in the differential diagnosis of anemias and other iron disorders. Iron deficiency anemia will have a low serum iron, a high TIBC, and a low percentage of transferrin saturation. Anemia of chronic disease will have a low serum iron, a low TIBC, and a normal percentage of transferrin saturation. In chronic disease, the body produces less transferrin. The Coombs test and other anti‐RBC antigen‐antibody assays (such as flow cytometry) should be considered if there is a high degree of suspicion for an immune‐mediated hemolytic anemia. Several diagnostic criteria can be used to make the diagnosis of an immune‐mediated hemolytic anemia and are listed in Table 10.6. Table 10.6 Diagnostic criteria for immune‐mediated hemolytic anemia. Not every parameter will be present in every patient. * For definitive diagnosis, one or more of the following hallmarks needs to be present [11]. Examination of the bone marrow is provided by aspiration and core biopsy to evaluate erythropoiesis. Obtaining bone marrow samples requires heavy sedation or anesthesia, with samples collected with an Illinois bone marrow needle or Jamshidi bone biopsy instrument. Common sites of collection include the humerus, tibia, femur, and wing of the ilium. Bone marrow aspirates and biopsy samples should be submitted along with a peripheral blood sample for CBC. Survey radiographs of the chest and abdomen are often the first imaging technique performed. Metallic (gastric) foreign objects are easily identified, with zinc, copper or lead known to cause anemia. Abdominal fluid is anticipated with loss of serosal detail, abdominal distension, splenic or hepatic masses or loss of detail in the retroperitoneal space. Abdominal ultrasound may help determine if there is free fluid in a body cavity (peritoneal, pleural, pericardial, or retroperitoneal) and may identify as a source of hemorrhage. Brief ultrasound examinations can be invaluable when searching for potentially life‐threatening cavitary hemorrhage. The abdominal focused assessment technique for trauma (AFAST) examines for free fluid in four areas of the abdomen: the diaphgramatic‐hepatic or subxiphoid view, the spleno‐renal or left flank view, the cysto‐colic view, and the hepato‐renal or right flank view. The AFAST technique can be used for trauma, as a triage tool, and to track the progress of the patient over time [12]. The thoracic focused assessment with sonography technique (TFAST) can be used to determine if there is pleural effusion or evidence of pulmonary hemorrhage or pericardial hemorrhage (see Chapters 8, 15 and Further reading). If blood is identified from either body cavity, a sterile centesis sample is obtained and the PCV or Hct and TP compared to the peripheral blood. Should significant volumes of blood be removed, the collected blood is saved in a sterile blood collection bag and available for autotransfusion if necessary. Video endoscopy (such as rhinoscopy, GI endoscopy, cystoscopy) may identify mucosal bleeding from ulcerative disease. Advanced imaging techniques such as computed tomography or magnetic resonance imaging may be helpful in select cases. Echocardiographic examination of patients with cardiac murmurs may be essential to help rule out underlying cardiac disease (see Chapter 11). Monitoring begins with the peripheral perfusion parameters (pulse rate and intensity, mucous membrane color, CRT) to assess the systemic effects of blood volume and the circulatory system. Blood pressure evaluation is important, with hypertension possible with renal disease and hypotension if severe anemia and hypovolemia (see Chapter 3). Body temperature and general physical and mental status are important to monitor during a blood transfusion, looking for signs of facial swelling, urticaria, vomiting, diarrhea, hyperpnea or shock. Observations should be made every five minutes for the first 20 minutes, every 15 minutes for the first hour and then hourly during initial assessment and resuscitation. Monitoring the PCV and TP is important for determining the trend of change in the amount of RBCs available for tissue oxygenation. The frequency is dependent upon the severity and progression of the illness. Many critically ill patients require multiple blood samples and the volume withdrawn should be kept to a minimum to prevent iatrogenic blood loss anemia. A variety of techniques are available to minimize blood loss: use of microhematocrit tubes, blood smear evaluation, three‐syringe collection techniques, and ear prick blood draws (Table 20.2). Patients receiving extracorporeal therapy such as dialysis are also at high risk of iatrogenic blood loss. If hemorrhage is a concern, monitoring the size or diameter of the area at risk can often detect continued blood loss before changes in perfusion become obvious. An assessment of the volume of blood loss should be made and the progression or resolution monitored. Diarrhea and vomit that resembles frank hemorrhage can be collected onto disposable pads and weighed. Fluids that can be collected from nasogastric tube suctioning can also be quantified. Fluid recovered from centesis and from various catheters (such as thoracostomy tube, urinary catheter, wound drains, peritoneal catheter), should be quantified and the PCV recorded. If the PCV of the fluid matches or is within 5 points of peripheral blood, it can be assumed that hemorrhage has occurred and concern for active, ongoing hemorrhage is warranted. When estimating surgical blood loss, blood‐soaked sponges, suctioned blood, bloody diarrhea/vomit, blood remaining on surgical equipment and drapes, and an estimate of removed blood clot volumes should all be included. A completely soaked 4 × 4 gauze can hold an average of 10 mL of blood [13,14]. Canisters that collect blood from a suction apparatus should be marked with the blood volume. Pulse oximetry measures the oxygen saturation of Hgb, not the quantity of Hgb. In patients with dyshemoglobinemia, pulse oximetry can give a falsely elevated reading, promoting a sense of false confidence. Co‐oximetry, which can measure carboxyhemoglobin and methemoglobin concentrations, is more useful (see Chapter 8). Increasing demands for oxygen delivery (DO2) occur when there is an increased rate of oxygen consumption (VO2). In the small animal ICU patient, an increase in VO2 can be a consequence of surgery, trauma, burns, inflammation, sepsis, pyrexia, shivering, seizures, agitation/anxiety/pain, adrenergic drugs, or even weaning from ventilation. The Hgb contained within the RBC is the main transporter of oxygen for distribution to the tissues. A deficit (anemia) or an excess (erythrocytosis) of RBCs will eventually affect blood flow and viscosity, resulting in insufficient DO2 to meet the demands of the cell. When left untreated, the resultant tissue hypoxia causes organ dysfunction and cell death. Identifying anemia or erythrocytosis is not the most difficult challenge; however, rapid recognition of potentially life‐threatening consequences of anemia or erythrocytosis is the initial focus. Resuscitation may be required prior to a definitive diagnosis of the underlying RBC disorder. Anemia is defined as a reduction in the oxygen‐carrying capacity of blood due to a decrease in Hgb concentration and RBC mass. Values used to diagnose anemia are PCV <37%, and Hgb <12 g/dL in the dog and PCV <27% and Hgb <9 g/dL in the cat. An algorithm to guide the initial decision‐making processes when managing the small animal ICU patient with anemia is provided in Figure 10.2. Figure 10.2 A decision‐making algorithm for the diagnosis and treatment of the small animal ICU patient with anemia. ABT, autologous blood transfusion; HBOC, hemoglobin‐based oxygen carrier; PCV, packed cell volume; pRBC, packed red blood cells; SIRS, systemic inflammatory response syndrome; TP, total protein; WB, whole blood. The presence of anemia will trigger important compensatory responses. To increase oxygen delivery to the tissues, the body will try to: (1) increase RBC or Hgb mass, (2) increase blood flow, and (3) increase oxygen unloading from Hgb in the capillaries [15]. These adaptations are possible due to mechanisms that increase cardiac output, redistribute blood flow, decrease the affinity of Hgb for oxygen, and increase oxygen extraction. The shift to the right of the oxyhemoglobin dissociation curve in anemia is primarily the result of increased production of 2,3‐diphosphoglycerate (2,3‐DPG) in the RBCs. This enables more oxygen to be released to the tissues at a given partial pressure of oxygen, offsetting the effect of the reduced oxygen‐carrying capacity of the blood. The dog has relatively high levels of red cell 2,3‐DPG with relatively high oxygen affinity. Increased 2,3‐DPG levels can be seen within four hours after significant hypoxia in the dog [16]. The cat, however, has low oxygen affinity hemoglobin that interacts weakly with 2,3‐DPG, and low concentrations of red cell 2,3‐DPG [17]. The most important determinant of the cardiovascular response to anemia is the blood volume status of the patient (left ventricular preload). Acute blood loss often results in anemia and hypovolemia and is termed hypovolemic anemia (see Figure 10.2). Clinical signs of hypovolemia may be noted when as little as 15% of blood volume is removed from healthy donor dogs [18]. With hypovolemic anemia, tissue hypoxia can occur from volume‐induced decreased cardiac output (circulatory hypoxia) as well as decreased oxygen‐carrying capacity (anemic hypoxia). The sympathetic nervous system redistributes the available cardiac output to vital organs by increasing arterial tone. The body will also initially increase RBC and Hgb mass through splenic contraction. The renin‐angiotensin‐aldosterone system is stimulated to retain water and sodium in an effort to increase blood volume. The loss of >15% of blood volume can result in increased heart rate (dogs) and decreased arterial blood pressure. Blood is rapidly shunted from the splanchnic, skeletal, and cutaneous organs towards the brain and the heart (see Chapter 2). An increase in cardiac output will also occur as a compensatory response to anemia when there is a normal blood volume, termed normovolemic anemia. An inverse relationship between the cardiac output and Hgb level exists as the Hgb declines below 12 g/dL [19]. Anemia causes a decrease in blood viscosity that reduces the resistance to blood flow. The net effect is an increase in blood flow to and from the heart (increased preload and decreased afterload). Increases in heart rate and myocardial contractility play only a minor role as long as normovolemia is maintained. Patients with normovolemic anemia may not have a change in blood pressure with the increase in cardiac output until anemia is severe. When severe, the heart rate will increase as well. At the systemic level, normovolemic anemia triggers a redistribution of blood flow to areas of high demand and with higher oxygen extraction ratios (heart, brain). At the microcirculatory level, three mechanisms may increase the amount of oxygen supplied to the tissues at the capillary networks: recruitment of previously closed capillaries, increased capillary flow, and increased oxygen extraction from existing capillaries [20]. There is decreased vascular resistance, which will increase blood flow, attributed to reduced inactivation of nitric oxide and an increased production of nitric oxide synthase [21]. As hemodilution increases, blood viscosity decreases, resulting in an increased rate of flow and a decrease in time spent in capillaries [22]. Blood viscosity is highest in postcapillary venules where flow is slowest. At this site, a disproportionate decrease in blood viscosity occurs as anemia worsens and, as a consequence, venous return is augmented for a given venous pressure [23,24]. When these mechanisms have increased to their maximum levels, further tissue hypoxia can result in signs of altered mentation, arrhythmias, and poor cardiac performance. The aggressive addition of fluids that do not contain RBCs or the inability to excrete water can cause a significant drop in the RBC count, PCV and Hgb concentration and intravascular volume overload. This is termed hypervolemic anemia. While there is a decrease in blood viscosity, there is also an additional demand on the cardiovascular system to handle the additional preload and vascular volume. An increase in glomerular filtration rate will enhance the excretion of excess water and electrolytes. Iatrogenic causes associated with fluid administration are first suspected in hypervolemic anemia in the ICU patient. However, underlying diseases such as liver cirrhosis, heart failure, acute oliguric renal failure, and increased antidiuretic hormone (such as head injury) can also cause hypervolemia and hemodilution. This form of anemia should be transient, with the restoration to a normal fluid balance the main mechanism of compensation. A recheck of the PCV and Hgb concentration is required when the fluid balance is corrected to evaluate for the persistence of anemia. Patients with hypovolemic anemia will require resuscitation of their cardiovascular system prior to or simultaneously with definitive treatment for the underlying cause of anemia. Emergent treatment is primarily directed at ensuring hemostasis, restoring RBC and Hgb concentrations, and safely reestablishing an adequate circulating blood volume. Providing supplemental oxygen during resuscitation can increase oxygen transport by ensuring that the blood Hgb is fully saturated and by raising the quantity of oxygen carried in solution in the plasma [25]. However, dissolved oxygen provides very little of resting tissue oxygen requirements and replacement of RBCs and Hgb is necessary. The baseline physical perfusion parameters (heart rate, pulse intensity, mucous membrane color, CRT) and blood pressure are assessed and 1–2 intravenous catheters placed for fluid resuscitation. Anemic animals in the decompensatory stage of shock or with ongoing, poorly controlled hemorrhage will require transfusion with whole blood (WB) or packed red blood cells (pRBCs) as part of the initial fluid resuscitation plan (see Blood transfusion below). A combination of isotonic balanced crystalloids and whole blood or crystalloids, synthetic colloid (such as hydroxyethyl starch) and pRBCs are infused to low‐normal resuscitation endpoints using small‐volume titration techniques (see Chapter 2). Fortunately, dogs do not have clinically significant preformed naturally occurring antibodies against the RBC antigens most often implicated in immediate hemolytic transfusion reactions (dog erythrocyte antigen (DEA) 1.1), making the first RBC transfusion a low risk for incompatibility but a high risk for sensitization. In cats, most fatal transfusion reactions are caused by incompatibilities in type B cats receiving type A RBCs. However, there may be more blood types in cats (such as Mik) that are not fully characterized. When blood is urgently needed, the risk of uncross‐matched RBCs must be weighed against the risk of delaying the transfusion. In the urgent setting, for dogs, DEA 1.1‐negative blood should be given if available. In both species, subsequent transfusions should be cross‐matched. A blood type is always recommended in cats [26]. If blood products are not available, and the patient has hemothorax or hemoabdomen, the blood retrieved from these cavities can be administered as an autologous blood transfusion (ABT). If hemoglobin‐based oxygen carrier (HBOC) solutions are available, the HBOC can be infused instead of the WB, pRBCs or ABT. The physical perfusion parameters and blood pressure are closely monitored throughout the resuscitation period. The patient history and physical examination are quickly assessed for indications of the possible source, severity, and longevity of hemorrhage. Providing hemostasis (when possible) becomes an immediate priority. A compression bandage may dampen the superficial flow of blood until definitive treatment can be provided. Ultimately, utilizing pressure to arrest hemorrhage depends on normal hemostatic mechanisms. Clamping with the tip of a hemostat for 3–5 minutes may be sufficient for small vessels. Large vessels will require ligation or electrocautery after local or general anesthesia. There are a variety of external and internal hemostatic agents available to assist in arresting hemorrhage. These hemostatic agents work through a variety of mechanisms: causing obstruction to blood flow, providing a framework for clotting to occur, absorbing water to increase effectiveness of natural clotting or stimulating the coagulation cascade; some of these are summarized in Table 10.7. Most of these products are intended for human use, and many are absorbable and do not need to be removed, but should be used with care in surgical wounds as they may inhibit healing [27]. Table 10.7 Examples of hemostatic agents by category [28,29]. Ongoing abdominal hemorrhage can be slowed or stopped by temporarily applying abdominal counterpressure, allowing a more detailed assessment and possible intervention once stabilized. It is important that the rear limbs are included in this bandage (Figure 10.3). The procedure for placing abdominal and hindlimb counterpressure is outlined in Box 10.5. Figure 10.3 Abdominal and hindlimb counterpressure.
Red blood cells and hemoglobin
Introduction
Diagnostic and monitoring methods
History
Drugs
Toxins
Oxidative
Acetaminophen (paracetamol)
Benzocaine
Propofol
Blood loss
Dapsone
NSAIDS
Anticoagulants
Aspirin, clopidogrel or other antiplatelet drugs or antithrombotic drugs
Glucocorticosteroids
Immune‐mediated hemolysis
Cephalosporins
Penicillins
Sulfonamides
Hemolysis
Fenbendazole
Heparin
Decreased marrow production
Amphotericin
Chloramphenicol
Cephalosporins
Fenbendazole
Griseofulvin
Phenobarbital
Phenothiazine
Recombinant human EPO
Sulfonamides
Oxidative
Crude oil
Naphthalene
Copper
Zinc
Propylene glycol
Blood loss
Dicoumarol
Anticoagulant rodenticides
Snakebite (DIC)
Hemolysis
Onions
Garlic
Decreased marrow production
Lead
Propylene glycol
Physical examination
Anemia
Erythrocytosis
Weakness
Pale mucous membranes (pallor)
Rapid or slow capillary refill time
Tachycardia
Altered pulses: bounding or weak
Decreased mentation
Lethargy
Tachypnea
Increased or decreased lung sounds
Heart murmur
Yellow mucous membranes (jaundice)
Pale or yellow skin color
Pigmenturia
Melena or frank blood on rectal exam
Epistaxis
Decreased size of ocular blood vessels
Pendulous abdomen or fluid wave
Absolute erythrocytosis
Red, injected or gray mucous membranes
Rapid (<1 sec) capillary refill time
Tachycardia
Bounding pulses
Heart murmur
Tachypnea
Injected/tortuous retinal vessels
Epistaxis
Hyphema
Gastrointestinal bleeding
Absent pulses, cold limbs (thrombosis)
Focal neurological deficits
Central neurological changes:
seizures, tremors, ataxia, blindness, behavior changes
Relative erythrocytosis
Clinical signs of dehydration
Clinical signs of hypovolemia
Hyperthermia (heatstroke)
Burns
Differential cyanosis (congenital)
Third body fluid spacing
Point of care testing
Parameter (units)
Definition
Dog normal
Cat normal
PCV(%)
Packed cell volume; volume of blood cells in a sample after it has been centrifuged in a hematocrit tube
35–57%
30–45%
TP (mg/dL)
Total protein; plasma protein
6.0–7.5
6.0–7.5
Hgb (g/dL)
Hemoglobin
11.9–18.9
9.8–15.4
RBC (×106/μL)
Red blood cell count
4.8–9.3
5.9–9.4
Hct (%)
Hematocrit; % of RBCs in blood; calculated from
MCV × RBC count/10
35–57%
30–45%
MCV (fL)
Mean corpuscular volume; measure of the average volume of a RBC
66–77
39–55
MCHC (g/dL)
Mean corpuscular hemoglobin concentration; measure of the concentration of hemoglobin in a given volume of packed RBCs
32.0–36.3
30–36
MCH (pg)
Mean corpuscular hemoglobin; measure of the average mass of hemoglobin in a RBC
21.0–26.2
13–17
Changes in PCV and TP
Possible etiology
↑ PCV ↑ TP
Dehydration
Polycythemia w/dehydration
Polycythemia w/hyperproteinemia
↑ PCV N TP
Polycythemia
Splenic contraction (stress)
↑ PCV ↓ TP
Blood loss (early) w/splenic contraction
Dehydration w/hypoproteinemia
N PCV ↓ TP
Acute hemorrhage w/splenic contraction
Hypoproteinemia
↓ PCV ↑ TP
Anemia w/dehydration
Anemia w/hyperproteinemia
↓ PCV N TP
Chronic anemia
↓ PCV ↓ TP
Acute blood loss
Anemia w/hypoproteinemia
Intravascular fluid overload
Blood smear
Finding
Description
Significance/etiologies
Spherocytosis
Lack of central pallor (dogs)
Primary or secondary immune‐mediated hemolytic anemia, recent blood transfusion, blood parasites, zinc toxicity, snake envenomation
Microcytosis
Small red blood cells
Iron deficiency (or chronic blood loss), portovascular anomalies, congenital or acquired, hyponatremia, normal in some breeds
Macrocytosis
Large red blood cells, may have concurrent polychromasia
Regenerative anemia, FeLV, myelodysplasia, hypernatremia
Hypochromasia
Pale color to cells
Chronic anemia
Polychromasia
Variation in cell color staining (pale, to normal to purple)
Regenerative anemia
Anisocytosis
Variation in cells size
Regenerative anemia
Keratocytes and shistocytes
Keratocytes are helmet shaped, shistocytes are fragments of RBCs
(i.e. half moon)
DIC, congestive heart failure, glomerulonephritis, myelofibrosis, hemangiosarcoma, doxorubicin toxicosis, burns
Acanthocytes
Irregularly spiculated cells
DIC, portovascular anomaly, chronic liver disease, lympohsarcoma, glomerulonephritis
Echinocytes
Even spacing between blunt to sharp projections from RBC membranes
Artifact, lymphosarcoma, glomerulonephritis
Heinz bodies
Single, light‐colored rounded protrusions of the RBC membrane
Onion, vitamin K, acetaminophen, methylene blue, propylene glycol, lymphosarcoma
Basophilic stippling
Small, dark blue inclusions
Lead poisoning
Nucleated RBCs
Dark purple nucleus in a normal‐sized RBC
Regenerative anemia, splenic disease, corticosteroids
Parasites that may be visualized
Babesia: large tear drop or ring shaped, often in pairs
Cytauxzoon: small, irregular intracellular rings
Hemobartonella canis: chain of small cocci or rods across the surface of RBCs.
Mycoplasma felis: nonrefractile cocci, rods or ring structures on periphery of RBCs
Babesia, Cytauxzoon, Mycoplasma, Hemobartonella infection
Saline agglutination
Clinicopathological laboratory testing
Diagnostic criteria
Methodology
Anemia
PCV/TP hematocrit or CBC with hemoglobin
Evidence of anti‐RBC antibodies
Gross or microscopic agglutination (see Box 10.3),* Coombs test,* flow cytometry
Evidence of RBC destruction
Spherocytosis,* hemolyzed serum, icterus (elevated total bilirubin), bilirubinuria or hemoglobinuria
Lack of other (primary) disease
Routine screening tests including thoracic and abdominal radiographs and/or abdominal ultrasound, screening for tick‐borne disease or other infectious, inflammatory or neoplastic disease
Response to therapy
Appropriate therapy and monitoring; may not be immediately evident
Evidence of regeneration
Polychromasia, anisocytosis, macrocytosis, hypochromasia, reticulocyte count, bone marrow analysis
Diagnostic imaging
Bedside monitoring techniques
Disorders caused by red blood cells
Anemia
Treatment of hypovolemic anemia
Category/mechanism
Examples
Manufacturer
Notes
Caustic agents.
Coagulating proteins, tissue necrosis, eschar formation
Aluminum Cl, ferric sulfate, silver nitrate, Zn Cl
Many manufacturers including Vet One (GF Health Products, Atlanta, GA), Kwik‐Stop (ARC Laboratories, Atlanta, GA)
Silver nitrate will cause pigmentation, Zn Cl is rarely used; often applied with an applicator stick or powder; external use only
Noncaustic Agents.
Primarily create a physical mesh facilitating platelet aggregation and amplification and propagation of coagulation
Gelatins; provide a matrix for clot formation, activated thrombin
Gelfoam (Pfizer, Memphis, TN),
Vetspon (Ethicon, Somerville, NJ)
Requires blood in field, not a sealing agent
Microporous polysaccharide hemispheres (MPH) from potato starch; hydrophilic and concentrate blood
Hemablock (Abbott, Abbott Park, IL)
Increase in volume of the spheres
Oxidized regenerated (methyl)cellulose (plant cellulose) – physical barrier for clotting, provides a matrix for clotting
Surgicell (Ethicon)
Acidic and therefore bactericidal
Microfiber collagen (bovine collagen) – aggregation and degranulation of platelets
Instat (Ethicon), Helistat (Integra, Plainsboro, NJ)
Wound should be dried and product applied with pressure using gauze
Physiological hemostatics (fibrin, thrombin, platelet gels, antifibrinolytics)
Aminocaproic acid (Hospira, Lake Forest, IL), others
Topical use may not be effective; little reported data
Fibrin glue – activates conversion of fibrinogen to fibrin
Tisseel/Tissucol (Baxter, Deerfield, IL), Hemaseel (Haemacure, Sarasota, FL), Evisel (Ethicon)
Dry field preferred, spray delivery
Glutaraldehyde adhesives – cross‐linking cell to cell proteins
Bioglue (Cryolife, Kennesaw, GA)
Dry field preferred
Waxes; malleable and form physical block as well as clotting surface
Bone wax (Aesculap, Center Valley, PA)
Difficult to apply to flat surface, may delay healing
Hemostatic dressings.
Used as wound dressings to prevent bleeding
Alginate dressing – seaweed protein, absorbs fluids through ion exchange reaction, releases calcium
Sorbosan (UDL Laboratories, Rockford, IL)
Not effective in high‐pressure bleeding nor for intracavitary use
Mineral zeolite – kaolin‐impregnated polyester gauze, kaolin immediately activates XII, XI; absorbs water
Quickclot (Z‐Medica, Wallingford, CT)
Foreign body reaction; early products were exothermic
Chitin (chitosan) dressing, from crab exoskeletons, adheres to and seal wounds
Celox gauze and powder (Medtrade, Crewe, UK)
May also be antimicrobial
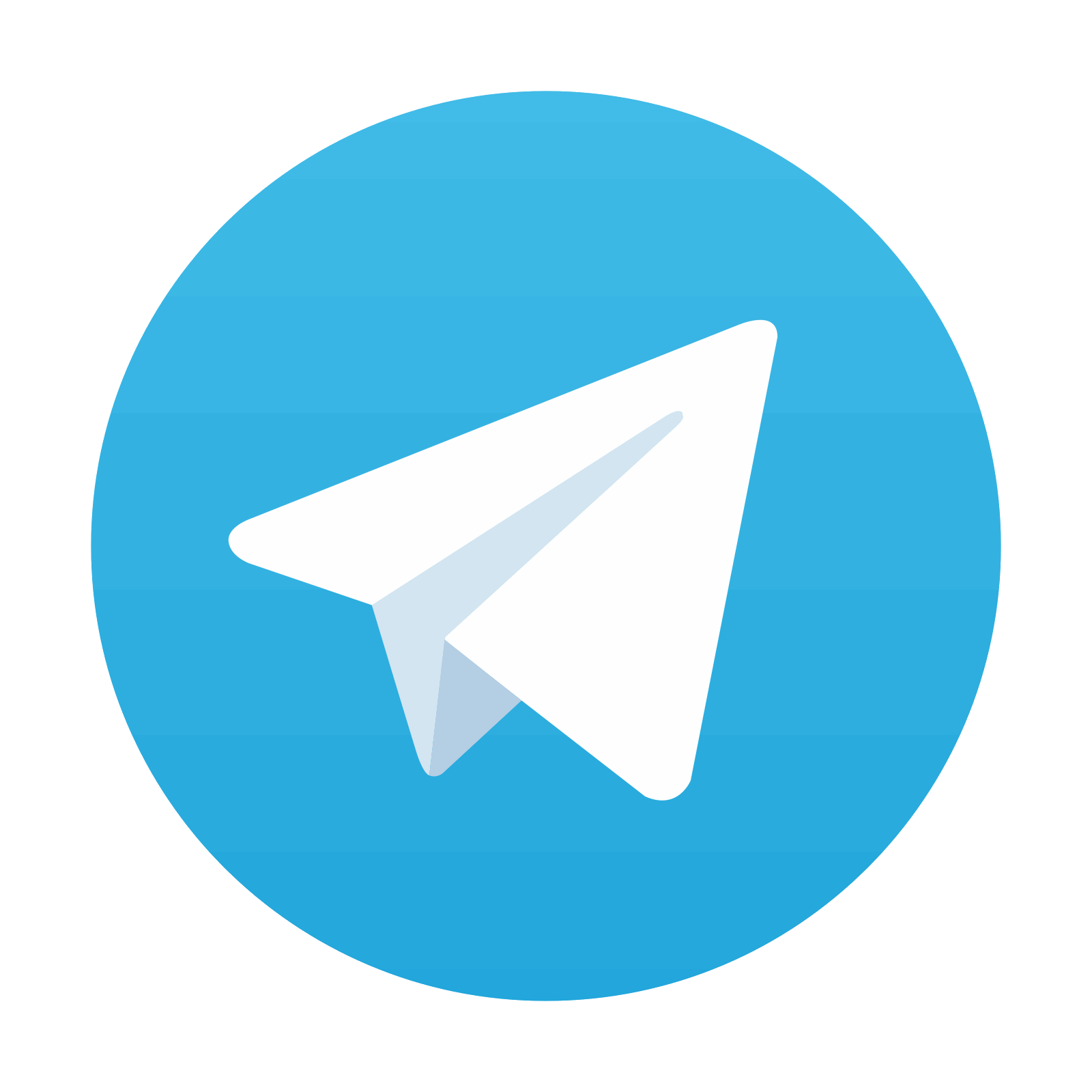
Stay updated, free articles. Join our Telegram channel

Full access? Get Clinical Tree
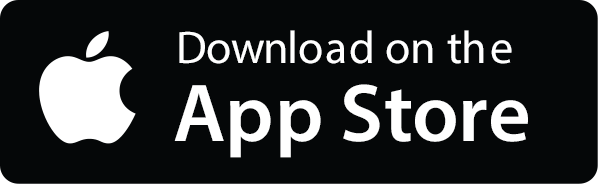
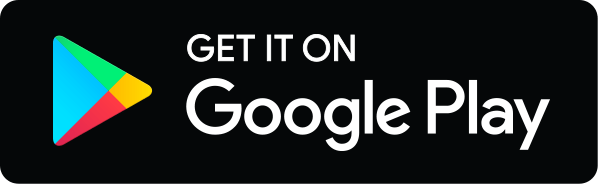