Figure 1.1Radiographs of healing radius and ulna fractures illustrating callus formation. (A) postoperative: cranial/ caudal view; (B) postoperative: lateral view. Plate osteosynthesis was performed with a DCP to allow dynamic compression. Note that the screws are located eccentrically away from the fracture line so that weight-bearing causes compression across the transverse fracture. The ulna was not repaired. The radius healed through primary bone union with minimal callus. The ulna healed with callus formation and is undergoing endochondral ossification.
The type of bone healing that is desired (primary or secondary) needs early consideration. Joint fractures should be rigidly and anatomically repaired (striving for primary bone union), so that callus or incongruity does not lead to a decreased range of motion and osteoarthritis. Areas of scant soft tissue coverage of bone or minimal endosteal blood supply are less likely to heal with secondary bone union, and should therefore be treated using techniques that encourage primary bone union. The treatment of distal radius and ulna fractures in small dogs are classic examples of when dynamic compression plating is preferential to buttress plating or external fixation.5 This is primarily due to the simple fracture configurations and the limited blood supply of this anatomical location. Interfragmentary compression can be achieved in these cases but the limited blood supply may be insufficient for secondary bone union.
Secondary bone union occurs most readily in specific fractures. For example, femoral, humeral, and pelvic fractures heal well by secondary bone union because of the abundant soft tissue coverage and robust blood supply. For these types of fracture, buttress plating with locking plates is often the treatment choice. Metaphyseal bone, which has rich vascularization, heals well by secondary bone healing. However, some fractures must heal by secondary bone union as anatomic alignment is not possible to allow primary bone union. Trying to anatomically reconstruct a highly comminuted femoral fracture, for example, frequently compromises normal healing mechanisms. This delays osteosynthesis and has an accompanying increased chance of infection and non-union.6
Age is a major consideration when evaluating the fracture patient.7 Juvenile bone heals much faster than adult bone, and geriatric patient bone can have delayed healing. If slower healing is anticipated, more rigid implants with a longer functional activity may be preferred. Ancillary procedures such as augmentation with bone grafting, cytokines, stem cells or platelet rich plasma (PRP) may be beneficial. Juvenile bone can also be softer than adult bone. Implants and devices that are weakly anchored in soft bone are less suitable than stronger constructs; locking screw plates and external fixators are often preferential to dynamic compression plates, for this reason.8, 9
Debilitated, often older, patients typically require more attentive treatment than younger, healthy patients. Specific attention should be given to appropriate nutrition and concomitant treatment of any pre-existing disease. Be aware however, that older patients tend to recover more quickly at home and all efforts should be made to discharge them from the hospital as soon as possible.
Patient size affects many aspects of bone healing, specifically in very large or very small patients. Very large patients may be too large for standard implants available for fracture repair in smaller-sized animals. For example, it would be inappropriate to use a 3.5 mm dynamic compression plate (DCP) for a tibia fracture in a dog weighing 100 kg, when the plate is used in buttress. Likewise, if a patient is very small it is tempting to use implants that are proportional. Remember that the area moment of inertia (AMI) for implants reflects non-linear increases in strength and stiffness as the dimensions of the implant increase. Surgeons often choose a screw or external fixator pin that is no greater than 20 percent of the bone diameter. As animals increase in size, their bones become relatively larger in linear proportion to their size and weight. However, the stiffness of increasingly larger implants is dictated by the AMI; which for a rod, is the radius to the fourth power (r4). Accordingly, the relative strength of an implant becomes greater as the implant becomes larger. Conversely, very small patients will require small implants and these are geometrically less stiff and weaker. As a consequence, comparatively larger implants may need to be chosen for smaller patients in order to achieve the same relative stiffness.
The normal activity levels of the patient should also be considered. More passive/docile patients will not place as much demand on fixation constructs as very active patients. Some young, active animals can ‘over-ride’ their concomitant physiologic pain to the detriment of their fixation repair. Again, these patients may have an anticipated need for a more robust fixation. Also, if they are very active or aggressive, constructs such as external fixators, that require frequent attention, may not be recommended or should be used with caution.
There are several owner considerations before determining a type of fracture repair. First, some owners are more compliant than others regarding patient care instructions. This includes exercise restriction as well as medications. A caregiver that is unable to closely monitor and care for their patient is less likely, for example, to accommodate an external fixator so internal fixation techniques should be favored. Additionally, appropriate routes of pain medication or antibiotics, with less frequent dosing intervals, would be more sensible.
Second, finance is often a significant issue for owners unexpectedly faced with a pet’s injury requiring surgery. The cost of implants vary and some implant costs are prohibitive. Although cost should not dictate repair technique, there are occasions when a simpler, less costly method can be successful even if not the optimal choice. For example, a pin and wire fixation of a spiral oblique tibial fracture is a fraction of the implant cost of a lag screw and locking plate repair.
Third, owner expectations need to be considered. For some performance animals, a return to full athletic function is desired. Other clients have less demanding expectations and will accept less than full function or some amount of disability. An excellent outcome for all repairs is unrealistic.
There may be permanent impairment of function and ultimately amputation may result in a better quality of life for the patient.
Fracture Assessment Implications for Healing and Implant Choice
First the surgeon should carefully assess the fracture by completing radiographic views, and a visual inspection and palpation of the fracture. Any assessment is performed systematically with consideration of the following.
- Assessing if the fracture is open or closed.
- Determining characteristics of the bone fracture and capacity for healing.
- Extent of the fracture within the bone (and joint).
- Kinetic energy associated with both the fractured bone and soft tissue damage.
- Evaluation of soft tissue coverage and soft tissue viability.
- Fracture displacement.
- Length of time from fracture occurrence to anticipated repair.
- General health of the patient.
The most commonly used classification system for open fractures is the Gustilo-Anderson classification.10, 11 This system aids prognosis of infection, non-union and other complication rates. It also provides a guide to treatment, antibiotic recommendations, timing for interventions and surgery. It enables a quick, consistent communication system for surgeons and researchers. Classification of fracture severity reflects the amount of energy, extent of soft tissue injury and the extent of contamination. Open fractures are classified as grades I–III. Open, grade III fractures are sub-classified as A, B, or C (see Table 1.1).
Table 1.1 Grade classification and sub-classification for open fractures
Grade | Description |
I | Open fracture, clean wound, wound <1 cm in length. |
II | Open fracture, wound >1 cm but < 10 cm, without extensive soft tissue damage, flaps, avulsions. |
III | Open with extensive soft-tissue laceration > 10 cm, damage or loss or an open segmental fracture. Fractures requiring vascular repair, or open fractures untreated for over 8 hours. |
Sub-classification of open grade III fractures | |
IIIA | Type III fracture with adequate periosteal coverage of the fracture bone despite extensive soft tissue damage. |
IIIB | Type III fracture with extensive soft tissue loss, periosteal stripping and bone damage. Massive contamination, need for soft tissue coverage procedure (flaps). An example of this type of fracture is seen in Figure 1.2. |
IIIC | Type III fracture associated with arterial injury requiring vascular repair. |
Although the classification system is helpful in communicating the severity of open fractures and generally dictating treatment, it is not very specific and clinicians are often in disagreement. Specifically the system does not clearly indicate the amount of energy imparted, nor any neurological damage or the degree of vascular compromise.
Grade I or II open fractures, when treated early, have little effect on prognosis or treatment choices. Treatment includes early and aggressive antibiotic treatment with a broad spectrum antibiotic. This is usually a first generation cephalosporin which should be administered as soon as possible parenterally and at the highest end of therapeutic doses; preferably within three hours of injury. If there is much contamination, an aminoglycoside should be added. Grade III open fractures should always be treated with a first generation cephalosporin and an aminoglycoside. In the case of contamination from heavy soil or fecal matter (for example, after farm injuries), metronidazole or clindamycin should also be administered for anaerobic coverage. The antibiotics should be administered for a maximum of 72 hours. Some surgeons discontinue antibiotics sooner; at the time of first debridement or soft tissue closure. A longer course of antibiotic therapy is not indicated and encourages antibiotic resistance. Infection rates have been reported as 2.3 percent for grade I and II fractures versus 17.6 percent for grade III.12
Figure 1.2 Photographs of an open, grade IIIB, highly comminuted, fracture of the diaphysis and distal metaphysis of the distal tibia. (A) preoperative image. Bone loss is present and the fracture included the distal articular surface. Although this limb was salvaged (B) postoperative image, the tibial tarsal joint was arthrodesed and lameness was always present.
Fracture location and configuration have a large impact on overall assessment and implant choice. The bone involved gives rise to considerations of both mechanics and biology. For example, surgical fixation of the pelvis has the advantage of a good blood supply and shorter bone levers acting on the fractures. Muscles of the pelvis often act to splint fractures. However, attention to impingement of the pelvic canal due to displacement, malunion, or large callus formation must still be considered. Femoral fractures have a good blood supply, but are subjected to large forces. The humerus has large and complex forces acting on it including bending and torsion. Fixation techniques that counter all fracture forces must be chosen to yield the best prognosis. The tibia has primarily axial loads and several basic repair implant devices are available. However, the distal tibia is the primary site for non-unions in humans and can be problematic in animals as well. Blood supply to the distal radius can be scant in small breed dogs which commonly results in delayed or non-unions.5 The metaphyseal region of many bones is rich in well-vascularized cancellous bone and has good healing potential which often allows for less rigid fixation methods. Salter fractures occur in the metaphyseal region of bones and have the advantage of a rich blood supply. By definition, Salter fractures occur in young animals associated with mechanically stable fracture configurations. In such cases, simple pin fixation can be successful, but must be applied to allow continued bone growth at the physes.
The amount of energy imparted to a fracture is an important consideration during assessment. The more energy imparted to the fracture, the larger amount of bone and soft tissue compromised. Gunshot wounds are good examples of this effect.13 A 0.22 long rifle round often has a 2.6 g lead slug and a muzzle velocity of 370 m/s with an energy of 141 J. By comparison, a 0.223 caliber (5.56 × 45 mm) round has a 2 g bullet, but a muzzle velocity of 1,140 m/s and an energy of 1.524 J. For a comparable size of slug, the second round has over ten times the energy of the first. In low velocity gunshot wounds, the fracture is treated essentially as a closed fracture.12 In contrast, high velocity gunshot wounds must be treated as grade III open fractures. These carry a much greater risk of infection, nonunion, and soft tissue damage. Nerve damage is frequent. The wound must be debrided and copiously lavaged. The wound is often left open.
Motor vehicle accidents impart considerable energy to bone and soft tissues depending on the velocity of the collision, size of patient, manner of impact, and whether the patient was run over by the vehicle. The degree of fracture comminution is an indicator of impact energy. Bone deformation is load-rate dependent; it bends differently based on the rate it is loaded. Bone is both viscoelastic and viscoplastic. If bone is loaded quickly, it is stiffer and will absorb more energy. When bone does fail, the energy is imparted throughout the loaded and deformed bone, resulting in more comminution. Bone loaded slowly tends to have more simple fracture characteristics and implies lower total energy imparted to the location. Also, the transfer of more energy from a high velocity fracture will result in more comminution. For these reasons, a highly comminuted fracture suggests a higher energy fracture and more trauma to both bone and soft tissue. Clinical consequences are less viable soft tissues which are more susceptible to infection and have a slower vascular recovery. There is subsequently retarded fracture healing. Skin and other soft tissues may die and need debridement. A higher energy fracture can be anticipated to require more time to heal, be more likely to require bone grafting either at the time of fixation or later during healing, and have a greater propensity for infection.
The skin of dogs and cats is relatively mobile so motor vehicle trauma can result in devitalized skin areas from tire pressure. Skin can therefore be devitalized with minimal energy absorption by the patient itself. Large areas of traumatized skin may subsequently die, requiring plastic procedures to close the defect. A patient completely run over by a vehicle often has considerable soft tissue injury. Vital structures inclusive within the urinary and gastrointestinal tracts may be injured and further compromise healing of fractures. A pelvic fracture with multiple fracture sites and comminution indicates an especially high-energy transfer.
The extent of soft tissue injury is often directly related to the energy of the injury; external forces great enough to fracture bone must first traverse through soft tissue. In contrast, fractures caused by a knife or axe (which are rare), may not have substantial associated soft tissue damage.
A fracture should be described using precise terminology and in a given sequence of descriptors. This allows accurate communication between clinicians and accurate medical records accuracy. The order of terms are:
- Whether the fracture is open or closed.
- The fracture configuration.
- Location of the fracture within the bone.
- The specific bone fractured.
- Displacement and over-riding presence.
A fracture is first described as open or closed and when open, its degree. The configuration of the fracture is then described; transverse, oblique, or comminuted. Transverse fractures are those perpendicular to the long axis of the bone, whereas other fractures would be oblique. Oblique fractures should be classified as short or long. An oblique fracture is defined as long when the length of obliquity is twice the diameter of the bone, and short when the obliquity is less than that. Differentiating between long and short oblique fractures significantly impacts on the choice of implant. All oblique fractures are subjected to shear forces during healing, and since most long-bone force is axial, the longer the obliquity, the greater the shear force.
Figure 1.3Radiographs of a closed, transverse fracture of the distal quarter of the diphysis of the radius, displaced laterally and over-riding 10 mm (A) preoperative: cranial/caudal view; (B) preoperative: lateral view.
Figure 1.4Radiographs of a grade II open, highly comminuted fracture of the mid diaphysis of the tibia, displaced laterally and over-riding 15 mm. (A) preoperative: cranial/ caudal view; (B) preoperative: lateral view.
Fracture location in a specific bone refers primarily to its metaphyseal or diaphyseal location. If metaphyseal, then the proximal or distal location should be noted. If the location is diaphyseal, then approximately which segment of the diaphysis (proximal quarter, mid-diaphyseal, or distal quarter) should also be noted.
The specific bone(s) fractured should then be noted. Logically, the bone in question would be noted first; however, the accepted custom is to first list the qualifiers above prior to identifying the specific bone. The next descriptor is displacement and over-riding (in millimeters) of the fracture. Always, the position of the distal fragment dictates the direction of displacement in orthogonal directions. These would be either cranial or caudal, then medial or lateral (compare Figures 1.3–1.6).
Figure 1.5Radiographs of a closed, Salter IV fracture of the distal humeral condyle. The capitulum is displaced laterally and over-riding 5 mm. (A) preoperative: cranial/caudal view; (B) preoperative: lateral view.
Figure 1.6Radiographs of a closed, long oblique fracture of the diaphysis of the humerus, displaced laterally and over-riding 2 cm. (A) preoperative: cranial/caudal view; (B) preoperative: lateral view.
Forces Acting on Bone and the Fracture
Normal bone is subjected to continual loads which are supported by means of forces. Changes to normal loads have to be countered by forces with equal and opposite force. These forces include tension (axial force), compression (axial force), shear, bending, and flexure, (torsion). It may be helpful to think of bone as a wall made up of bricks and mortar (see schematics shown in Figures 1.7–1.10). The bricks represent the mineral component of bone and the mortar represents collagen. An axial load is the load produced by pushing on the ends of long bones. The direction of gravity as well as the structure of the skeletal system and pull of muscles results in long bones being subjected to loads orientated along the long axial, hence, these are referred to as axial loads. One can liken this to a force pushing down on the wall (Figure 1.7).
Compression loads are typically much larger than other loads. As such, bone is able to counter relatively large axial force. Fixation constructs must be equally stout in providing optimal strength and stiffness to counter these forces to enable bone healing.
Tension or tensile forces are those that distract a bone. They separate the joint surfaces without rupture, for example, as facilitated by the attachment of muscles by tendons. Using the wall analogy again, tensile forces are those that would lift a brick off the wall. Whilst bone can sustain large compressive forces, far less force is required to pull apart a section of bone (Figure 1.8).
Figure 1.7Schematic illustrating analogy of bone as a brick wall and the effects of forces acting on it. The bricks represent the mineral component and the mortar, the collagen. Such a structure is very strong under axial loads due to compression forces (indicated by the direction of the blue arrow).
Shear forces are those exerting opposite directional, but unaligned, forces on a structure. If these forces were directly opposite in alignment, they would be compressive. Since they are not aligned, these forces tend to cause a shifting or sliding of one part of a structure against another part of the same structure. Shear forces can disrupt bone healing14 and can be thought of, in the context of the brick wall analogy, as pushing on one section of the brick and mortar wall and having one group of bricks slide along another group, as shown in Figure 1.9.
Bending is the result of a combination of both compressive and tensile forces. As a cylindrical beam is bent, for example, tensile forces act on one side of the beam and compressive forces on the opposite side. In the center, there will be no compressive or tensile forces. This is called the neutral plane. There will be various directions and magnitudes of compressive and tensile forces throughout the structure due to bending, resulting in shear stresses between adjacent areas. Bone is best able to accommodate compressive forces, less able to accommodate tensile forces, and quite poor at accommodating shear forces. Accordingly, in fractures subjected to bending, a partially transverse fracture in the area of bone subjected to tension is accompanied by obliquity or fragmentation from shear forces in the area of bone subjected to compression (Figure 1.10). Using the wall analogy again, pushing at the top of the wall would result in the mortar separating on the side of the wall that was being pushed. If ever asked to knock down a wall, the easiest method would be by using a bending force.
Figure 1.8Schematic illustrating how traction is a tensile force on bone (blue arrow indicates force direction) (A). Fractures due to tension forces are usually simple and transverse (B).
Figure 1.9Schematic illustrating that shear forces are opposite in direction but occur in the same plane (blue arrows indicate force directions) (A). Bone is relatively weak with regard to its ability to withstand the stress imposed by shear forces (B).
Figure 1.10
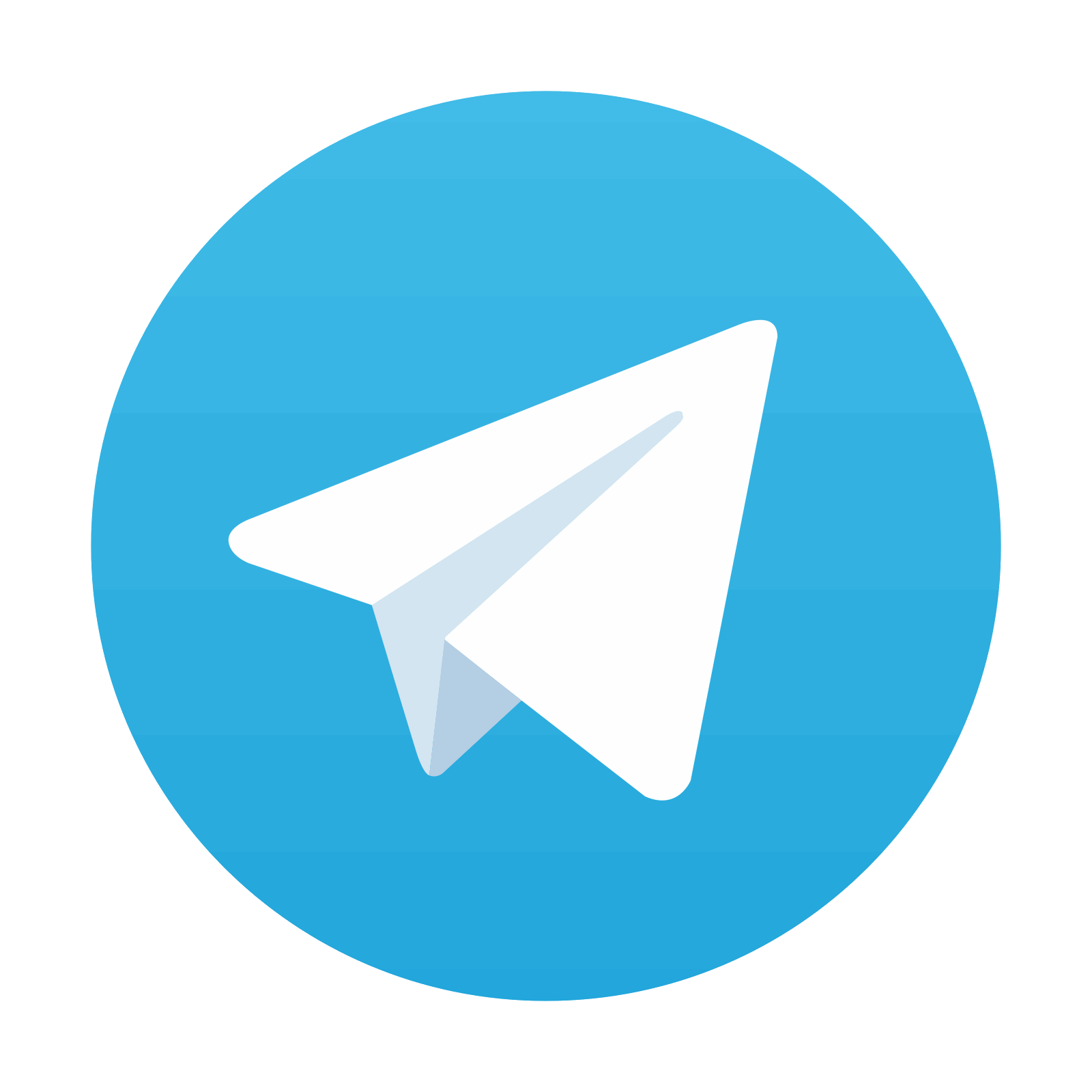
Stay updated, free articles. Join our Telegram channel

Full access? Get Clinical Tree
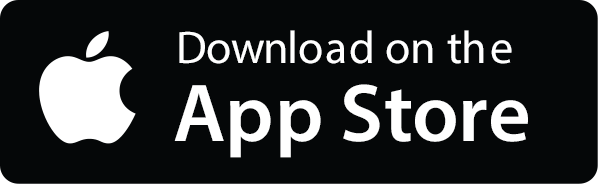
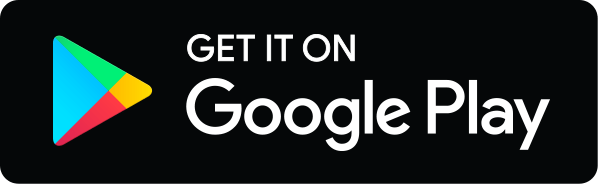