CHAPTER 1 Rebecca Kirby (Formerly) Animal Emergency Center, Gainesville, Florida Heat stroke, peritonitis, parvovirus diarrhea, systemic lymphosarcoma, leptospirosis, massive trauma, gastric dilation‐torsion, aspiration pneumonia, pancreatitis, immune‐mediated disease, and postoperative laparotomy are but a sampling of the multitude of potentially life‐threatening disorders that can affect the small animal intensive care unit (ICU) patient. These and other disorders share a common pathophysiology: an inciting stimulus initiates the production and release of circulating mediators that cause systemic inflammatory changes. Inflammation can be defined as a localized protective response elicited by injury or destruction of tissues that serves to destroy, dilute, or wall off both the injurious agent and the injured tissue [1]. Chemical mediators are released in response to an inciting antigen and initiate the innate immune response that causes inflammation. The classic signs of inflammation are heat, redness, swelling, pain, and loss of normal function. These are manifestations of the physiological changes that occur during the inflammatory process: (1) vasodilation (heat and redness), (2) increased capillary permeability (swelling), and (3) leukocytic exudation (pain). The initial inflammatory response to a localized insult is good, serving to localize the problem, destroy an offending pathogen, clean up damaged tissues, and initiate the healing process. However, many ICU patients develop a negative trajectory when the inflammatory mediators and their response have systemic consequences. When this occurs due to an infection, it is called sepsis, and when it progresses, it often results in multiple organ dysfunction syndrome (MODS) or multiple organ failure (MOF). It might appear logical that an overwhelming infectious agent could stimulate systemic inflammation. Yet, an almost identical clinical progression has been commonly observed in response to conditions that are not due to infection (such as trauma, surgery, and certain metabolic diseases). The term “sepsis syndrome” was first used to describe this in human patients when they appeared to be septic but had no obvious source of infection [2–4]. By the mid‐1990s, sepsis syndrome had evolved into the nomenclature of systemic inflammatory response syndrome (SIRS). It was discovered that the body can respond to noninfectious insults and tissue injury in the same exaggerated manner that it does to microbial pathogens, with an almost identical pathophysiology [5]. In sepsis, pathogen‐associated molecular patterns (PAMPs), expressed by the pathogen, stimulate pattern recognition receptors (PRRs) in the host. With noninfectious diseases, damaged tissues also release endogenous mediators, such as alarmins and damage‐associated molecular pattern (DAMP) molecules (such as heat shock proteins, HMGB‐1, ATP, and DNA). These will stimulate the toll‐like receptor, PRRs or other receptor systems that typically respond to microbes and activate immune cell responses [6–8]. A list of proinflammatory cytokines associated with SIRS is provided in Table 1.1. Figure 1.1 provides a schematic of many of the proinflammatory changes that occur in this syndrome. Table 1.1 Inflammatory and hemostatic mediators of severe sepsis and their effects. Adapted from: Balk RA, Ely EW, Goyette RE. Stages of infection in patients with severe sepsis. In: Sepsis Handbook, 2nd edn. Thomson Advanced Therapeutics Communication, 2004, pp 24–31. Figure 1.1 A schematic of some of the major consequences of the proinflammatory component of systemic inflammatory response syndrome (SIRS). Many cells produce proinflammatory mediators, including monocytes, macrophages, and endothelial cells. The interaction of an antigen (microbial or tissue based) with its receptor will cause the stimulation of protein kinase C and the production of cytokines. Cytokines in the circulation will interact with their specific receptor on other cells and stimulate the production of more cytokines. In addition to the release of cytokines (IFN, IL‐1, TNF), the arachidonic acid cascade is stimulated and produces PG, PAF, and leukotrienes. Reactive oxygen species are produced as well. Some of the consequences include degranulation of white cells, endothelial damage, stimulation of coagulation, white blood cell chemotaxis and adherence in capillaries, and increased phagocytosis of Ags. Ag, antigen; IFN, interferon; IL, interleukin; O2., superoxide radicals; PAF, platelet activating factor; PG, prostaglandins; TNF, tumor necrosis factor; TLR, toll‐like receptor; TTP, tissue thromboplastin; VIIa, activated factor VII. Soon the one‐hit and two‐hit models of MODS caused by SIRS were recognized in humans; one hit results from an initial massive insult (traumatic, metabolic, infectious), culminating in early SIRS and MODS. The two hits occur when a severely injured patient is successfully resuscitated, followed by a second inflammatory insult which amplifies SIRS and results in MODS [9,10]. It was discovered that an antiinflammatory response occurred after the initial inflammatory response as well. This compensatory antiinflammatory response syndrome (CARS) is characterized by increased appearance of antiinflammatory cytokines and cytokine agonists found in the circulation [11]. These antiinflammatory mediators were found for days or weeks after the proinflammatory mediators had gone [12]. Macrophage dysfunction is a significant contributor to CARS, with a decreased capacity to present antigens and release proinflammatory cytokines [13]. It was found that the T‐cells are defective and depleted due to apoptosis and decreased proliferation [14]. In addition, there is an increase in the suppressor cell populations [15]. Many of the cytokines released during CARS are listed in Table 1.1. Figure 1.2 provides a schematic of many of the antiinflammatory changes that occur during this process. Figure 1.2 A schematic of some of the major consequences of the antiinflammatory component of the compensatory antiinflammatory response syndrome (CARS). Red dotted lines depict inhibitory actions, blue solid lines depict stimulatory action. T‐cells, monocytes, and macrophages are the primary cells affected. The same antigens (microbial or tissue based) that stimulate the proinflammatory response can also stimulate the antiinflammatory cascades. The antiinflammatory mediators will block the production of many of the proinflammatory cytokines (red triangle and red dotted lines). TNF and IL‐1 receptors are found in the circulation and will bind and inactivate TNF and IL‐1 proinflammatory mediators. Ag, antigen; IFN, interferon; IL, interleukin; IL‐1R, interleukin‐1 receptor; iNOS, inducible nitric oxide synthetase; O2., superoxide radicals; PD‐1, programmed death‐1; TGF‐β, tissue growth factor‐beta; TLR, toll‐like receptor; TNF, tumor necrosis factor; TNFR, tumor necrosis factor receptor. It was determined that the production of proinflammatory and antiinflammatory cytokines occurs simultaneously, with antiinflammatory gene expression paralleling the increased expression of proinflammatory genes [16]. It was then proposed that the induction of SIRS and CARS occurs simultaneously [17]. The emergence of myeloid‐derived suppressor cells (MDSCs) results in suppression of T‐cell responses through increased production of nitric oxide and reactive oxygen species. The increase in MDSCs is proportional to the severity of the inflammatory insult [17]. Although the pathophysiology has not been clearly defined for the SIRS‐CARS phenomenon, the basic hemodynamic consequences have been identified. Once the mediators have entered the circulation, the progression and complications are similar for each inciting disease: peripheral vascular dilation, increased capillary permeability, and depressed cardiac function. Three forms of shock are known to occur simultaneously in these patients: hypovolemic, distributive, and cardiogenic (see Figure 1.3). Once shock ensues, MODS is likely to occur if aggressive patient support has been delayed. Figure 1.3 A schematic depicting the presence of proinflammatory (blue) and antiinflammatory (yellow) mediators released concurrently (green), causing hemodynamic changes that result in three simultaneous forms of shock. Many research and clinical trials have been conducted in laboratory animals and humans looking for a single best therapy that would be effective in treating most patients with the SIRS‐CARS phenomenon, with minimal success. Since inflammation and immune suppression have been found to be occurring simultaneously, each patient is more likely to be experiencing their own unique combination of immune stimulation and suppression. This makes a standardized protocol for therapy extremely difficult to formulate until further knowledge is acquired. Emphasis is no longer primarily directed at methods to stop exaggerated proinflammatory responses but is instead placed on supporting the patient and searching for new methods that prevent prolonged immunosuppression or restore immune function [18]. Sepsis, the SIRS‐CARS phenomenon (referred to simply as SIRS from here on), and MODS remain tremendous obstacles to the successful treatment of critically ill small animals. A “back to basics” approach is critical for any patient with the potential for inflammatory changes. Several basic yet key principles that can be used to guide patient assessment and care are listed in Box 1.1. Problems within the major organ systems should be anticipated in advance, with appropriate diagnostic, therapeutic, and monitoring efforts employed early, rather than waiting for a problem to surface and reacting to it. The Rule of 20 was developed to assist the critical care team in thoughtfully and carefully assessing these patients. Table 1.2 lists common problems to anticipate under each parameter of the Rule of 20 in patients with SIRS. Sample forms that can be used when applying the Rule of 20 are provided in Figures 1.4 and 1.5. Table 1.2 Common problems to anticipate for each parameter of the Rule of 20 in the SIRS patient. COP, colloidal osmotic pressure; DIC, disseminated intravascular coagulation; TLC, tender loving care; WBC, white blood cell. Figure 1.4 The Rule of 20. Each parameter should be assessed regularly in any critically ill dog or cat. The order of importance will vary between individual patients. COP, colloidal osmotic pressure; GI, gastrointestinal, TLC, tender loving care; WBC, white blood cell. Figure 1.5 Rule of 20 form for recording current patient status, targeted endpoints, and proposed intervention. RBC, red blood cell; WBC, white blood cell.
An introduction to SIRS and the Rule of 20
Introduction to the Rule of 20 and inflammatory response syndromes
Proinflammatory mediators
Tumor necrosis factor
IL‐6 induction, TF expression, downregulation of TM gene expression and increased catabolism, activation of fibrinolysis, cytotoxicity, upregulation of endothelial cell adhesion molecules, induction of NO synthase, neutrophil activation, antiviral activity, fever, and other effects; circulating soluble receptor is antagonist
Interleukin‐1
Fever, synthesis of acute‐phase proteins, induction of IL‐6 synthesis, upregulation of TF expression, decreased TM expression, activation of fibrinolysis, and other effects
Interleukin‐6
Induction of acute‐phase response, induces B‐cell growth and T‐cell differentiation, enhances NK‐cell activity, promotes maturation of megakaryocytes, can inhibit endotoxin‐induced IL‐1 and TNF‐alpha; circulating soluble receptor is agonist
Interleukin‐8
Release stimulated by TNF, IL‐1, IL‐2, promotes chemotaxis, enhances neutrophil function, upregulates adhesion molecule expression, level correlates with severity of systemic manifestation of pathology
Interferon‐gamma
Induction of IgG production, potentiation of activity of IL‐12, macrophage activation
Antiinflammatory mediators
Interleukin‐4
Stimulation and inhibition of various classes of T‐cells, suppression of TNF and IL‐1 secretion, upregulation of IgE and IgG secretion
Interleukin‐10
Inhibition of inflammatory cytokine production by mononuclear cells, suppression of monocyte procoagulant activity, downregulation of monocyte killing, share receptor homology with interferon
Transforming growth factor‐beta
Tissue development and repair, other antiinflammatory properties
Soluble receptor and receptor antagonists
Soluble TNF‐1 receptor and IL‐1 soluble receptor inhibit function
Hemostatic factors
Tissue factor
Upregulates expression on monocytes and subset of endothelial cells by TNF and IL‐1 leading to stimulation of extrinsic coagulation cascade
Thrombin
Protein C
Protein S
Antithrombin
Plasminogen activator inhibitor‐1
Tissue factor pathway inhibitor
Plasmin
Thrombin activatable fibrinolysis inhibitor
Other mediators
Nitric oxide
Bradykinin
Lipopolysaccharide binding protein
Complement
Leukotrienes
Prostaglandins
Superoxide radicals
Platelet activating factor
Myocardial depressant factor
Rule of 20 parameter
Anticipated problems
Fluid balance
Hypovolemia; vasodilation, increased capillary permeability
Albumin, COP
Hypoalbuminemia; loss through capillaries and catabolic state; reduced COP and extravasation of fluid from vasculature
Blood pressure
Hypotension; hypovolemia and impaired cardiac performance, peripheral vasodilation
Glucose
Hypoglycemia; increased consumption, decreased intake; can affect vascular tone and cardiac performance
Electrolytes
Hypokalemia; any disorder is possible with fluid imbalances, catabolic state, and depressed nutritional intake
Acid–base
Metabolic acidosis associated with poor perfusion and elevated lactate is common
Oxygenation/ventilation
Hypoxemia possible if pulmonary edema from inflammatory vascular changes or poor cardiac performance
Coagulation
Thrombocytopenia (declining trend); some form of DIC due to mediator‐induced vasculitis, activation of serine proteases, and insufficient antithrombin
Red blood cells
Anemia from disease or erythrocytosis from dehydration; frequent blood sampling can result in anemia
Heart rate, rhythm, contractility
Tachycardia in dogs with poor perfusion, bradycardia in cats with poor perfusion; arrhythmias if poor perfusion; depressed myocardial contractility with circulating mediators
Neurological status
Depressed level of consciousness from perfusion changes, hypoxemia if present, circulating mediators, metabolic changes or underlying disease
Urinary tract status
Azotemia if poor perfusion or dehydration; impaired renal function if prolonged hypotension or nephrotoxic drugs
WBC, immune status
Impaired immune function, lymphopenia possible, susceptible to nosocomial infections
Gastrointestinal status
Gastric paresis, ileus; third body fluid spacing into bowel; bacterial translocation if no enteral feeding
Nutrition
Catabolic state and early malnutrition anticipated; bacterial translocation without enteral feeding
Drugs
Altered volume of distribution, metabolism and excretion
Body temperature
High if active inflammation; low in cats with poor perfusion or dogs with difficult‐to‐resuscitate shock
Pain control
Pain is anticipated with all critical illness and deserves analgesic support; critical early in shock resuscitation
Wound and bandage care
Possible source of pathogens requiring close monitoring of wound sites and bandage changes
Nursing care and TLC
Must receive the right treatment at the right time in the right amount; anticipate problems, be ready; provide TLC
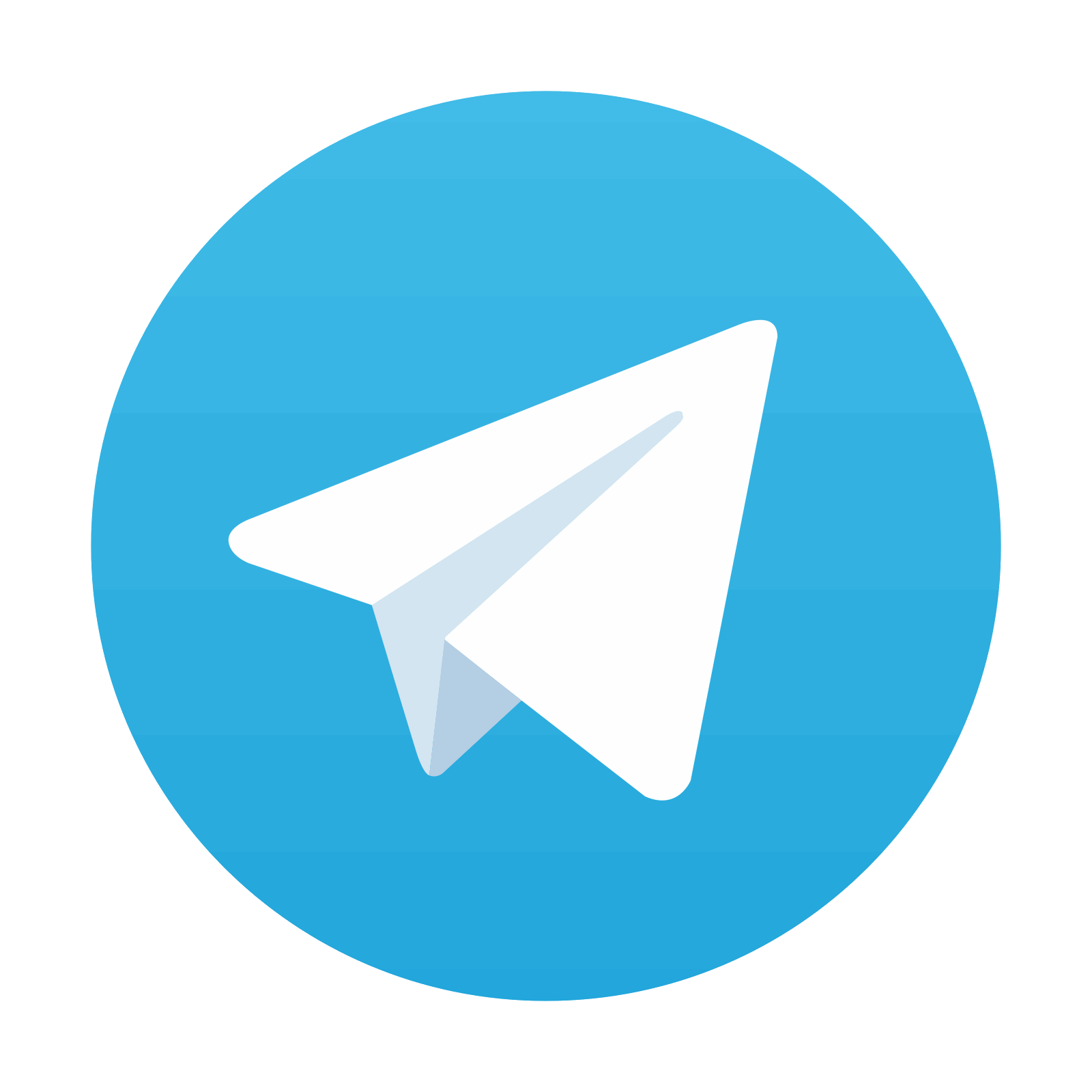
Stay updated, free articles. Join our Telegram channel

Full access? Get Clinical Tree
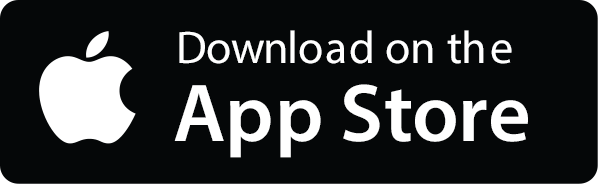
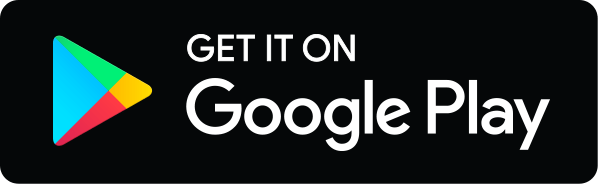